Nanolasers
Introduction
Nanolasers are a class of lasers that have dimensions on the nanometer scale. These devices, which are at the intersection of photonics and nanotechnology, have the potential to revolutionize fields as diverse as computing, communication, and medicine. This article will delve into the principles, fabrication, and applications of nanolasers, as well as the challenges and future prospects of this exciting technology.
Principles of Operation
Nanolasers operate on the same basic principles as larger lasers. They generate light through the process of stimulated emission, where an incoming photon of a specific energy can cause an excited atom or molecule to drop to a lower energy state, emitting a second photon in the process. This photon is identical to the incoming one in terms of phase, frequency, polarization, and direction. When this process is sustained and amplified within a resonant cavity, a coherent beam of light is produced.
The key difference between nanolasers and their larger counterparts lies in the size of the resonant cavity. In a nanolaser, the cavity dimensions are on the order of the wavelength of the light being emitted, which leads to unique quantum mechanical effects. One of these is the confinement of the electromagnetic field, which can enhance the interaction between light and matter, leading to a higher rate of stimulated emission and thus a lower threshold for lasing.
Fabrication
The fabrication of nanolasers is a complex process that requires precise control over material properties and dimensions at the nanoscale. The most common methods involve top-down approaches such as photolithography and electron beam lithography, where a larger piece of material is carved down to the desired dimensions. These methods can produce structures with high precision, but they are often expensive and time-consuming.
Another approach is the bottom-up method, which involves assembling the nanolaser from individual atoms or molecules. This can be achieved through techniques such as chemical vapor deposition (CVD) or molecular beam epitaxy (MBE). These methods can potentially produce nanolasers with superior properties, such as higher quality factor and lower threshold, but they also present challenges in terms of control and reproducibility.
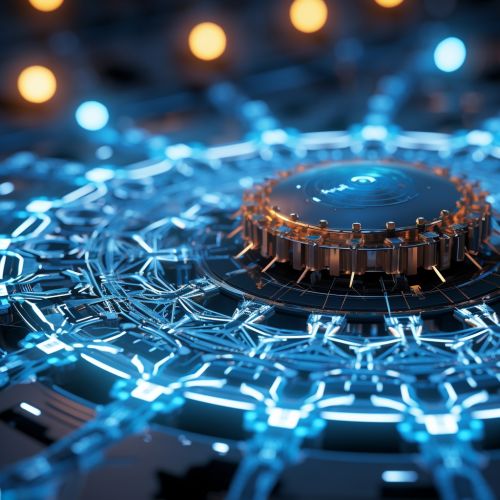
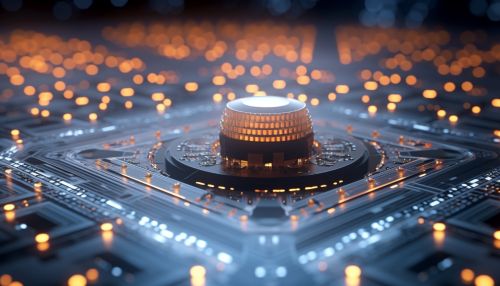
Applications
Nanolasers have a wide range of potential applications, thanks to their small size, low power consumption, and unique optical properties.
In the field of optical computing, nanolasers could be used to create ultra-fast and energy-efficient processors. By replacing electronic transistors with optical ones, data could be processed at the speed of light, leading to a significant increase in computing power.
In telecommunications, nanolasers could enable the development of ultra-compact and high-speed optical communication devices. These could be used to transmit data over long distances with minimal loss, or to interconnect electronic components within a single chip.
In biomedical engineering, nanolasers could be used for imaging and therapy at the cellular or even molecular level. For example, they could be used to illuminate specific targets within a cell, or to selectively destroy cancerous cells without harming the surrounding healthy tissue.
Challenges and Future Prospects
Despite the exciting potential of nanolasers, there are several challenges that need to be addressed. One of the main issues is the high threshold for lasing, which is caused by the small size of the resonant cavity and the resulting high losses. This can be mitigated by improving the quality factor of the cavity, but this often comes at the expense of other properties, such as the emission wavelength or the output power.
Another challenge is the integration of nanolasers with other components, such as waveguides and detectors, to create functional devices. This requires precise alignment and coupling, which can be difficult to achieve at the nanoscale.
Despite these challenges, the future of nanolasers looks promising. With advances in fabrication techniques and a better understanding of the underlying physics, it is expected that the performance of nanolasers will continue to improve, opening up new possibilities for their application.