Quantum Dot Laser
Quantum Dot Laser
A **quantum dot laser** is a type of semiconductor laser that utilizes quantum dots as the active laser medium. Quantum dots are nanoscale semiconductor particles that have quantum mechanical properties, which allow them to emit light at specific wavelengths when excited. This unique characteristic makes quantum dot lasers highly efficient and tunable, with applications in telecommunications, medical diagnostics, and quantum computing.
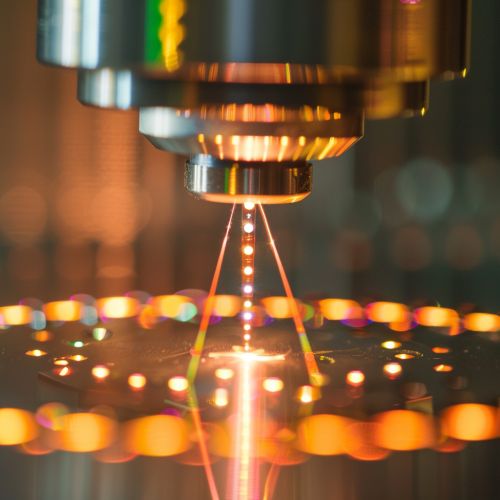
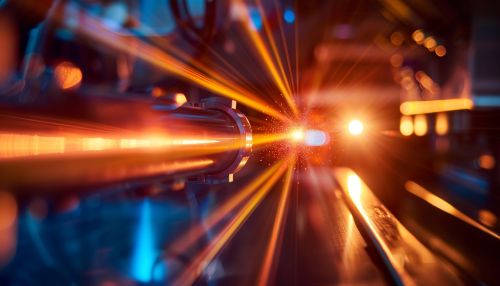
Historical Background
The concept of quantum dots was first introduced in the 1980s by Alexei Ekimov and Louis Brus, who independently discovered that semiconductor nanoparticles could exhibit quantum confinement effects. The development of quantum dot lasers began in the 1990s, with significant contributions from researchers such as Yasuhiko Arakawa and Hiroyuki Sakaki. Their work demonstrated that quantum dots could be used to create lasers with lower threshold currents and higher temperature stability compared to traditional semiconductor lasers.
Quantum Dots
Quantum dots are semiconductor nanocrystals typically ranging from 2 to 10 nanometers in diameter. Due to their small size, quantum dots exhibit discrete energy levels, similar to atoms, which is why they are sometimes referred to as "artificial atoms." The energy levels of quantum dots can be tuned by changing their size, shape, and material composition, allowing for precise control over the emitted light's wavelength.
The most common materials used for quantum dots include cadmium selenide (CdSe), indium arsenide (InAs), and lead sulfide (PbS). These materials are chosen for their ability to form stable nanocrystals with well-defined quantum confinement properties.
Operating Principles
Quantum dot lasers operate based on the principles of quantum confinement and stimulated emission. When an external energy source, such as an electrical current or optical pump, excites the quantum dots, electrons are promoted from the valence band to the conduction band, creating electron-hole pairs known as excitons. These excitons recombine, releasing energy in the form of photons. The emitted photons can stimulate the emission of additional photons from other excitons, leading to a coherent light output.
The key advantage of quantum dot lasers is their ability to achieve low threshold currents and high efficiency. This is because quantum dots have a high density of states at the band edge, which enhances the probability of radiative recombination. Additionally, the three-dimensional confinement of carriers in quantum dots reduces the sensitivity of the laser's performance to temperature variations.
Fabrication Techniques
The fabrication of quantum dot lasers involves several steps, including the synthesis of quantum dots, their incorporation into a semiconductor matrix, and the formation of the laser cavity. Common methods for synthesizing quantum dots include colloidal synthesis, molecular beam epitaxy (MBE), and metal-organic chemical vapor deposition (MOCVD).
1. **Colloidal Synthesis**: This method involves the chemical reaction of precursors in a solution to form quantum dots. It allows for precise control over the size and composition of the quantum dots and is suitable for large-scale production.
2. **Molecular Beam Epitaxy (MBE)**: MBE is a vacuum-based technique that deposits atomic layers of materials onto a substrate. Quantum dots are formed by the self-assembly of deposited atoms under specific conditions. MBE offers high purity and control over the quantum dot's properties.
3. **Metal-Organic Chemical Vapor Deposition (MOCVD)**: MOCVD is a chemical vapor deposition technique that uses metal-organic precursors to grow thin films and quantum dots on a substrate. It is widely used in the semiconductor industry for its scalability and compatibility with existing fabrication processes.
After synthesizing the quantum dots, they are embedded into a semiconductor matrix, typically made of materials like gallium arsenide (GaAs) or indium phosphide (InP). The quantum dot-embedded matrix is then processed to form the laser cavity, which includes mirrors or distributed Bragg reflectors (DBRs) to provide optical feedback and enhance the laser's output.
Applications
Quantum dot lasers have a wide range of applications due to their unique properties, including:
1. **Telecommunications**: Quantum dot lasers are used in fiber-optic communication systems for their high efficiency and tunability. They enable the transmission of data over long distances with minimal signal loss and distortion.
2. **Medical Diagnostics**: In medical diagnostics, quantum dot lasers are employed in fluorescence imaging and biosensing. Their narrow emission spectra and high brightness make them ideal for detecting specific biomolecules and imaging cellular structures.
3. **Quantum Computing**: Quantum dot lasers play a crucial role in the development of quantum computing technologies. They are used to generate single photons and entangled photon pairs, which are essential for quantum information processing and communication.
4. **Display Technology**: Quantum dot lasers are also being explored for use in display technology, such as quantum dot light-emitting diodes (QD-LEDs). These displays offer higher color purity and energy efficiency compared to traditional display technologies.
Advantages and Challenges
- Advantages**:
- **Low Threshold Current**: Quantum dot lasers require lower threshold currents to achieve lasing, making them more energy-efficient. - **Temperature Stability**: The performance of quantum dot lasers is less affected by temperature variations, ensuring consistent operation across a wide temperature range. - **Wavelength Tunability**: The emission wavelength of quantum dot lasers can be precisely tuned by adjusting the size and composition of the quantum dots. - **High Modulation Speed**: Quantum dot lasers can achieve high modulation speeds, making them suitable for high-speed data transmission.
- Challenges**:
- **Fabrication Complexity**: The synthesis and incorporation of quantum dots into semiconductor matrices require precise control and advanced fabrication techniques, which can be complex and costly. - **Material Stability**: Some quantum dot materials, such as cadmium-based compounds, can be toxic and unstable, posing challenges for long-term reliability and environmental safety. - **Integration with Existing Technologies**: Integrating quantum dot lasers with existing semiconductor technologies and manufacturing processes can be challenging, requiring significant research and development efforts.
Future Directions
The future of quantum dot lasers lies in overcoming the current challenges and expanding their applications. Researchers are exploring new materials and fabrication techniques to improve the stability and performance of quantum dot lasers. Additionally, efforts are being made to integrate quantum dot lasers with silicon photonics, enabling the development of compact and efficient photonic circuits for various applications.
Advancements in quantum dot laser technology are also expected to drive innovations in quantum computing and communication, paving the way for more secure and efficient information processing systems. Furthermore, the development of environmentally friendly and non-toxic quantum dot materials will enhance the sustainability and safety of quantum dot lasers, making them more viable for widespread use.