Atomic Force Microscopy
Introduction
Atomic Force Microscopy (AFM) is a high-resolution imaging technique that falls under the umbrella of Scanning Probe Microscopy. It was first developed in 1986 by Gerd Binnig, Calvin Quate, and Christoph Gerber. AFM is a powerful tool in the field of nanotechnology, allowing scientists to visualize and manipulate individual atoms and molecules with unprecedented precision.
Principle of Operation
The operation of an AFM is based on the interaction between a sharp probe (the tip) and the sample surface. The tip is located at the end of a cantilever, which is typically made of silicon or silicon nitride. The cantilever is designed to respond to the forces between the tip and the sample surface, which can include van der Waals forces, electrostatic forces, magnetic forces, and others.
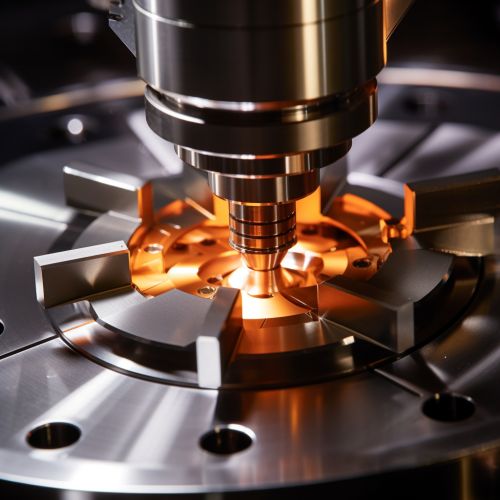
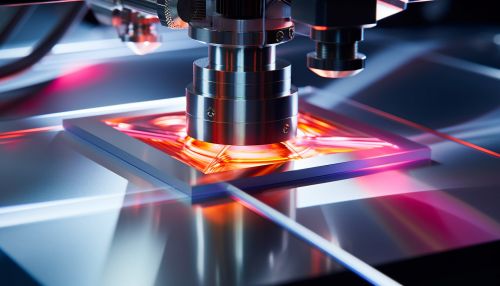
The deflection of the cantilever is measured using a laser beam that is reflected off the back of the cantilever and onto a position-sensitive photodetector. The photodetector records the position of the laser spot, which changes as the cantilever deflects. This information is used to generate a topographic map of the sample surface.
Modes of Operation
There are several modes of operation for an AFM, including contact mode, non-contact mode, and tapping mode.
In contact mode, the tip of the AFM is in constant contact with the sample surface. This mode provides high-resolution images, but it can also cause damage to the sample or the tip due to the physical contact.
In non-contact mode, the tip oscillates above the sample surface without making contact. This mode is less damaging to the sample and the tip, but it provides lower resolution images.
Tapping mode, also known as intermittent contact mode, is a compromise between contact and non-contact modes. In this mode, the tip oscillates and periodically touches the sample surface. This mode provides high-resolution images with less damage to the sample and the tip.
Applications
AFM has a wide range of applications in various fields, including physics, chemistry, biology, materials science, and nanotechnology.
In physics and chemistry, AFM is used to study the surface properties of materials at the atomic level. It can provide information about the topography, friction, magnetic properties, and other characteristics of a sample.
In biology, AFM is used to image and manipulate biological samples, such as proteins, DNA, and cells. It can also be used to measure the mechanical properties of biological materials.
In materials science, AFM is used to study the properties of various materials, including polymers, ceramics, composites, and metals. It can provide information about the surface roughness, hardness, and other properties of a material.
In nanotechnology, AFM is used to manipulate individual atoms and molecules, which is essential for the development of nanoscale devices and materials.
Limitations
Despite its many advantages, AFM also has some limitations. For example, it can only image the surface of a sample, not its interior. It also has a limited scan size, typically up to 150 micrometers in the x and y directions and up to 10 micrometers in the z direction.
Furthermore, AFM requires a flat sample surface for optimal imaging. Rough or uneven surfaces can cause the tip to crash into the sample, damaging the tip and the sample.
Finally, AFM imaging can be time-consuming, especially for large samples. It can take several minutes to several hours to acquire a single image, depending on the scan size and the imaging speed.
Future Developments
Despite these limitations, AFM continues to be a valuable tool in many fields of research. Future developments in AFM technology are likely to improve its speed, resolution, and versatility.
For example, high-speed AFM is a recent development that allows for real-time imaging of dynamic processes at the nanoscale. This could open up new possibilities for studying biological processes, chemical reactions, and other dynamic phenomena.
Furthermore, advances in tip technology could improve the resolution of AFM. For example, the use of carbon nanotube tips could potentially allow for atomic-resolution imaging.
See Also
Scanning Electron Microscopy Transmission Electron Microscopy Scanning Tunneling Microscopy