Superconducting Nanowires
Introduction
Superconducting nanowires are a class of superconducting materials that have dimensions on the nanometer scale. These materials exhibit unique properties due to their reduced dimensions and the effects of quantum mechanics. Superconducting nanowires are used in a variety of applications, from quantum computing to sensitive detectors for electromagnetic radiation.
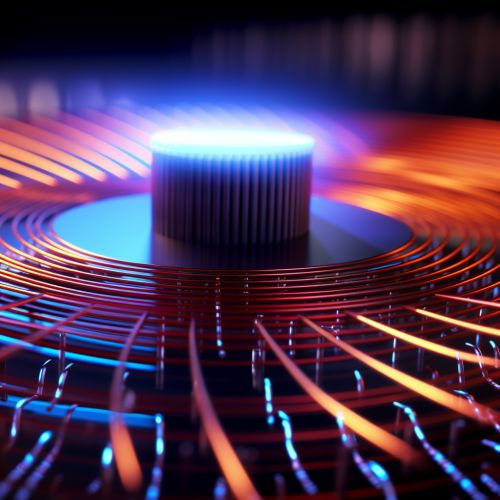
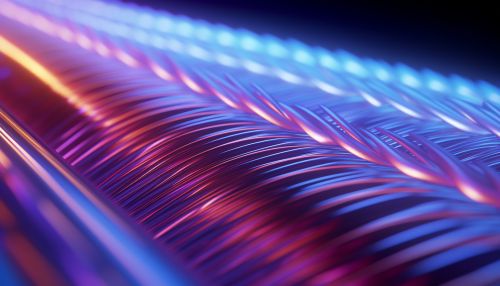
Properties of Superconducting Nanowires
Superconducting nanowires exhibit several unique properties that distinguish them from bulk superconductors. These properties result from the interplay of quantum mechanics and the reduced dimensions of the nanowires.
Quantum Effects
Quantum effects become significant in superconducting nanowires due to their small dimensions. One such effect is the quantum confinement, which leads to the discretization of the energy levels in the nanowire. This can result in an increase in the critical temperature, the temperature below which the material becomes superconducting.
Critical Current
The critical current, the maximum current that a superconducting nanowire can carry without resistance, is also affected by the nanowire's dimensions. As the diameter of the nanowire decreases, the critical current also decreases. This is due to the increased influence of surface effects and the reduced number of conduction channels in the nanowire.
Proximity Effect
The proximity effect is another important property of superconducting nanowires. When a superconducting nanowire is in contact with a normal metal, the superconducting properties can penetrate into the normal metal. This effect can be used to induce superconductivity in non-superconducting materials.
Fabrication of Superconducting Nanowires
Superconducting nanowires can be fabricated using several techniques, including lithography, chemical vapor deposition, and molecular beam epitaxy. The choice of fabrication technique depends on the desired properties of the nanowire, such as its dimensions, material composition, and crystal structure.
Lithography
Lithographic techniques are commonly used to fabricate superconducting nanowires. These techniques involve the use of a mask to pattern a resist layer on a substrate, followed by etching to remove the exposed areas of the substrate. The remaining resist can then be removed to leave behind the desired nanowire structure.
Chemical Vapor Deposition
Chemical vapor deposition (CVD) is another technique used to fabricate superconducting nanowires. In CVD, a volatile precursor is decomposed on a heated substrate to form a solid material. By controlling the conditions of the deposition, nanowires with specific properties can be grown.
Molecular Beam Epitaxy
Molecular beam epitaxy (MBE) is a technique used to grow high-quality superconducting nanowires. In MBE, a beam of atoms or molecules is directed onto a substrate, where they condense to form a solid. By carefully controlling the conditions of the beam and the substrate, nanowires with precise dimensions and crystal structures can be grown.
Applications of Superconducting Nanowires
Superconducting nanowires have a wide range of applications, from quantum computing to sensitive detectors for electromagnetic radiation.
Quantum Computing
In quantum computing, superconducting nanowires are used to create qubits, the basic units of quantum information. Superconducting qubits have several advantages over other types of qubits, including long coherence times and the ability to be integrated into large-scale quantum circuits.
Single-Photon Detectors
Superconducting nanowires are also used to create single-photon detectors, devices that can detect the presence of a single photon. These detectors are used in a variety of applications, including quantum communication, quantum cryptography, and astronomical observations.
Superconducting Magnetic Energy Storage
Superconducting nanowires can also be used in superconducting magnetic energy storage (SMES) systems. In these systems, a superconducting coil is used to store energy in the magnetic field. The energy can then be released when needed by allowing the coil to return to its normal state.