Cuprate superconductor
Introduction
Cuprate superconductors are a family of high-temperature superconductors that contain copper-oxide planes. These materials exhibit superconductivity at temperatures much higher than conventional superconductors, making them a significant area of research in condensed matter physics. The discovery of cuprate superconductors has revolutionized the field of superconductivity and opened new avenues for technological applications.
History
The discovery of cuprate superconductors dates back to 1986 when physicists J. Georg Bednorz and K. Alex Müller discovered superconductivity in a lanthanum barium copper oxide (LaBaCuO) compound at a temperature of 35 K. This breakthrough earned them the Nobel Prize in Physics in 1987 and sparked intense research into high-temperature superconductors.
Structure and Composition
Cuprate superconductors are characterized by their layered structure, consisting of copper-oxide (CuO2) planes separated by layers of other elements. The general formula for these compounds is RBa2Cu3O7−δ, where R is a rare earth element such as yttrium or one of the lanthanides. The copper-oxide planes are crucial for superconductivity, as they are the primary sites for electron pairing.

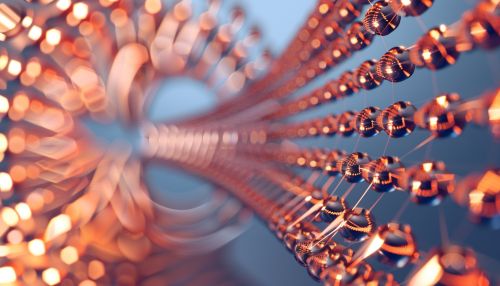
Mechanism of Superconductivity
The mechanism of superconductivity in cuprates is not fully understood, but it is believed to involve unconventional pairing of electrons. Unlike conventional superconductors, which are explained by the BCS theory, cuprates exhibit d-wave pairing symmetry. This means that the electron pairs, or Cooper pairs, have a different angular momentum compared to s-wave pairing in conventional superconductors.
Electron Correlation
One of the key features of cuprate superconductors is the strong electron correlation within the copper-oxide planes. This correlation leads to complex electronic behavior, including the formation of a pseudogap phase, where the density of states is partially suppressed. Understanding these correlations is crucial for unraveling the mechanism of high-temperature superconductivity.
Types of Cuprate Superconductors
Cuprate superconductors can be classified into several families based on their chemical composition and structure. Some of the most well-known families include:
Yttrium Barium Copper Oxide (YBCO)
YBCO, with the chemical formula YBa2Cu3O7−δ, is one of the most studied cuprate superconductors. It has a critical temperature (Tc) of around 93 K, making it a prime candidate for practical applications. YBCO is also known for its high critical current density and magnetic field tolerance.
Bismuth Strontium Calcium Copper Oxide (BSCCO)
BSCCO compounds, such as Bi2Sr2CaCu2O8+δ, are another important family of cuprate superconductors. These materials have a layered structure with multiple copper-oxide planes, leading to higher Tc values, sometimes exceeding 100 K. BSCCO is often used in the fabrication of superconducting tapes and wires.
Thallium Barium Calcium Copper Oxide (TBCCO)
TBCCO compounds, such as Tl2Ba2Ca2Cu3O10, are known for their very high Tc values, sometimes reaching up to 125 K. These materials are less commonly used due to the toxicity of thallium, but they remain an important area of research.
Applications
Cuprate superconductors have a wide range of potential applications due to their high Tc values. Some of the most promising applications include:
Power Transmission
High-temperature superconducting (HTS) cables made from cuprate superconductors can transmit electricity with zero resistance, reducing energy losses and increasing efficiency. These cables are particularly useful in urban areas where space is limited.
Magnetic Resonance Imaging (MRI)
Cuprate superconductors are used in the construction of high-field MRI magnets. These magnets provide better image resolution and faster scanning times compared to conventional MRI systems.
Particle Accelerators
Superconducting magnets made from cuprate superconductors are used in particle accelerators to generate strong magnetic fields. These magnets are essential for guiding and focusing particle beams in experiments such as those conducted at the LHC.
Challenges and Future Directions
Despite their potential, cuprate superconductors face several challenges that need to be addressed for widespread adoption. These include:
Material Synthesis
The synthesis of high-quality cuprate superconductors is complex and requires precise control over composition and structure. Advances in material science are needed to develop more efficient and scalable synthesis methods.
Understanding the Mechanism
A complete understanding of the mechanism of superconductivity in cuprates remains elusive. Further theoretical and experimental research is needed to uncover the underlying principles and guide the development of new materials.
Stability and Durability
Cuprate superconductors are often sensitive to environmental factors such as humidity and temperature. Improving the stability and durability of these materials is crucial for their practical applications.