BCS Theory
Introduction
The BCS theory, named after its creators John Bardeen, Leon Cooper, and John Robert Schrieffer, is a theoretical framework that explains how electrical resistance in certain materials disappears at extremely low temperatures, a phenomenon known as superconductivity. The theory, proposed in 1957, is a cornerstone in the field of condensed matter physics and has been instrumental in advancing our understanding of quantum mechanics and solid-state physics.
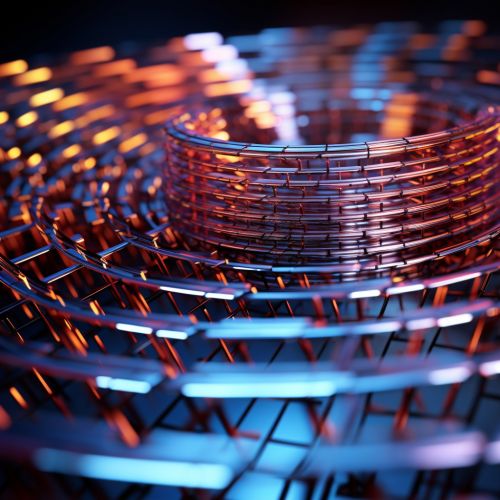
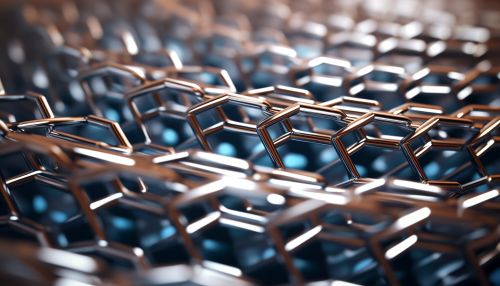
Fundamentals of BCS Theory
The BCS theory is built on the concept of Cooper pairs, named after Leon Cooper, one of the theory's co-creators. These pairs consist of two electrons with opposite momenta and spins, which form at low temperatures in certain materials, known as superconductors. The formation of Cooper pairs is a quantum mechanical effect and is the key to understanding the phenomenon of superconductivity.
Cooper Pair Formation
In a normal conductor, electrons move independently and their motion is impeded by impurities and lattice vibrations, leading to electrical resistance. However, in a superconductor at low temperatures, a remarkable thing happens. An electron moving through the lattice can attract nearby positive ions, creating a region of higher positive charge density. This region can then attract a second electron, forming a Cooper pair.
The pairing mechanism is mediated by phonons, quanta of lattice vibrations. The interaction between electrons and phonons leads to an attractive force between two electrons, overcoming their natural repulsion and allowing them to form a pair. This pairing process is a many-body effect and cannot be understood in terms of interactions between individual electrons.
Energy Gap and Superconducting State
The formation of Cooper pairs leads to a fundamental change in the energy structure of the superconductor. An energy gap is created between the ground state, which is filled with Cooper pairs, and the excited states. This energy gap is directly related to the binding energy of the Cooper pairs and is a key feature of the BCS theory.
The superconducting state is characterized by a macroscopic quantum state where all Cooper pairs occupy the same quantum state. This state is a quantum mechanical phase of matter and is distinct from the normal conducting state. The transition between the normal state and the superconducting state is a phase transition, similar to the transition between liquid and gas.
Implications and Applications of BCS Theory
The BCS theory has far-reaching implications and has found numerous applications in various fields of physics and engineering. It has provided a theoretical foundation for the development of superconducting technologies, such as superconducting magnets used in MRI machines and particle accelerators.
Quantum Mechanics and Condensed Matter Physics
The BCS theory is a significant achievement in the field of quantum mechanics. It is one of the few many-body problems in quantum mechanics that has been solved exactly. The theory has also played a crucial role in the development of condensed matter physics, providing insights into the behavior of electrons in solids.
Technological Applications
Superconductivity, as explained by the BCS theory, has numerous technological applications. Superconducting materials, due to their zero electrical resistance, are used in power transmission lines, magnetic levitation trains, and superconducting quantum interference devices (SQUIDs). The theory has also been instrumental in the development of Josephson junctions, which are used in superconducting quantum computing.
Limitations and Future Directions
While the BCS theory has been remarkably successful in explaining low-temperature superconductivity, it has its limitations. It fails to explain high-temperature superconductivity, observed in certain materials known as cuprates and iron-based superconductors. The mechanism of high-temperature superconductivity remains one of the major unsolved problems in physics.
Future research in the field of superconductivity is aimed at understanding the mechanism of high-temperature superconductivity and finding new superconducting materials. The discovery of room-temperature superconductivity, which could revolutionize technology, remains a tantalizing goal.