Quantum confinement: Difference between revisions
(Created page with "== Quantum Confinement == Quantum confinement refers to the phenomenon that occurs when the dimensions of a material are reduced to a size comparable to the de Broglie wavelength of the electrons, typically in the nanometer range. This reduction in size alters the electronic and optical properties of the material due to the spatial restriction of the charge carriers. Quantum confinement is a critical concept in the field of nanotechnology, particularly in the st...") |
No edit summary |
||
Line 3: | Line 3: | ||
Quantum confinement refers to the phenomenon that occurs when the dimensions of a material are reduced to a size comparable to the [[de Broglie wavelength]] of the electrons, typically in the nanometer range. This reduction in size alters the electronic and optical properties of the material due to the spatial restriction of the charge carriers. Quantum confinement is a critical concept in the field of [[nanotechnology]], particularly in the study of [[quantum dots]], [[nanowires]], and [[quantum wells]]. | Quantum confinement refers to the phenomenon that occurs when the dimensions of a material are reduced to a size comparable to the [[de Broglie wavelength]] of the electrons, typically in the nanometer range. This reduction in size alters the electronic and optical properties of the material due to the spatial restriction of the charge carriers. Quantum confinement is a critical concept in the field of [[nanotechnology]], particularly in the study of [[quantum dots]], [[nanowires]], and [[quantum wells]]. | ||
[[Image:Detail-92341.jpg|thumb|center|A close-up image of quantum dots under a microscope, showing their distinct colors due to quantum confinement effects.|class=only_on_mobile]] | |||
[[Image:Detail-92342.jpg|thumb|center|A close-up image of quantum dots under a microscope, showing their distinct colors due to quantum confinement effects.|class=only_on_desktop]] | |||
=== Principles of Quantum Confinement === | === Principles of Quantum Confinement === |
Latest revision as of 23:57, 14 June 2024
Quantum Confinement
Quantum confinement refers to the phenomenon that occurs when the dimensions of a material are reduced to a size comparable to the de Broglie wavelength of the electrons, typically in the nanometer range. This reduction in size alters the electronic and optical properties of the material due to the spatial restriction of the charge carriers. Quantum confinement is a critical concept in the field of nanotechnology, particularly in the study of quantum dots, nanowires, and quantum wells.
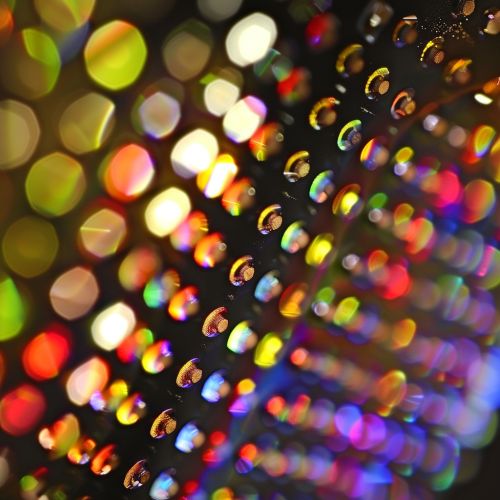
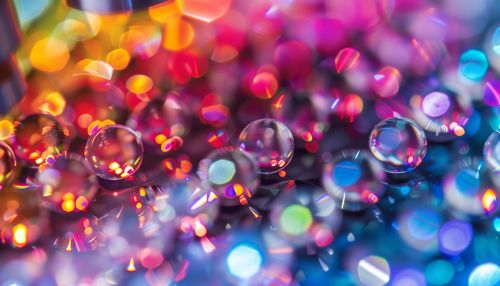
Principles of Quantum Confinement
Quantum confinement arises when the motion of electrons or holes is restricted to a dimension that is comparable to their de Broglie wavelength. In bulk materials, electrons and holes are free to move in three dimensions, and their energy levels form continuous bands. However, when the material is confined to a lower dimension, the energy levels become quantized, leading to discrete energy states.
The degree of confinement can be classified into three regimes:
- **Strong Confinement:** When the size of the material is smaller than the exciton Bohr radius, leading to significant quantization effects.
- **Weak Confinement:** When the size is larger than the exciton Bohr radius but still within the nanometer scale, resulting in moderate quantization.
- **Intermediate Confinement:** When the size is comparable to the exciton Bohr radius, leading to a mix of bulk and quantum behaviors.
Quantum Dots
Quantum dots are semiconductor nanoparticles that exhibit quantum confinement in all three spatial dimensions. These nanocrystals have unique optical and electronic properties that differ significantly from their bulk counterparts. The size of the quantum dot determines the energy levels and, consequently, the color of light they emit when excited. This size-dependent emission makes quantum dots highly valuable in applications such as biological imaging, solar cells, and light-emitting diodes (LEDs).
Quantum Wells
A quantum well is a potential well that confines particles in one dimension, allowing free movement in the other two dimensions. Quantum wells are typically created by sandwiching a thin layer of a semiconductor material between two layers of a material with a larger band gap. This structure leads to the quantization of energy levels in the confined dimension, which can be exploited in devices such as quantum well lasers and high-electron-mobility transistors (HEMTs).
Quantum Wires
Quantum wires are structures that confine electrons or holes in two dimensions, allowing free movement along the remaining dimension. These one-dimensional systems exhibit unique transport properties, such as ballistic transport and quantum conductance. Quantum wires are used in various applications, including nanoelectronics and spintronics.
Effects of Quantum Confinement
The primary effects of quantum confinement include:
- **Energy Level Discretization:** The continuous energy bands of bulk materials become discrete energy levels, leading to unique optical and electronic properties.
- **Band Gap Increase:** The band gap of the material increases as the size decreases, resulting in a blue shift in the absorption and emission spectra.
- **Enhanced Carrier Mobility:** Confinement can lead to reduced scattering and increased carrier mobility, beneficial for electronic and optoelectronic devices.
- **Modified Density of States:** The density of states changes from a continuous distribution to discrete levels, affecting the material's electronic and optical behavior.
Applications of Quantum Confinement
Quantum confinement has numerous applications across various fields:
- **Optoelectronics:** Quantum dots and wells are used in LEDs, lasers, and photodetectors due to their tunable optical properties.
- **Biological Imaging:** Quantum dots are employed as fluorescent markers in biological imaging, offering higher brightness and stability compared to traditional dyes.
- **Solar Cells:** Quantum dots enhance the efficiency of solar cells by enabling multiple exciton generation and broad-spectrum absorption.
- **Quantum Computing:** Quantum dots and wells are explored as potential qubits for quantum computing due to their discrete energy levels and coherence properties.
Challenges and Future Directions
Despite the promising applications, several challenges remain in the practical implementation of quantum confinement:
- **Synthesis and Fabrication:** Achieving precise control over the size, shape, and composition of nanostructures is critical for consistent quantum confinement effects.
- **Stability and Reliability:** Ensuring the long-term stability and reliability of quantum-confined materials in various environments is essential for commercial applications.
- **Integration with Existing Technologies:** Integrating quantum-confined materials with existing semiconductor technologies requires overcoming compatibility and scalability issues.
Future research is focused on addressing these challenges and exploring new materials and structures that exhibit quantum confinement. Advances in nanofabrication techniques and a deeper understanding of quantum mechanics will drive the development of next-generation devices and applications.