Quantum wires
Introduction
Quantum wires are one-dimensional nanostructures that exhibit quantum mechanical properties. These structures are typically fabricated from semiconductor materials and have diameters on the order of nanometers, which is comparable to the de Broglie wavelength of electrons in the material. This confinement leads to quantization of the electronic energy levels, resulting in unique electrical, optical, and thermal properties that are distinct from those of bulk materials or even two-dimensional systems like quantum wells.
Fabrication Techniques
The fabrication of quantum wires involves advanced techniques to achieve the necessary nanoscale dimensions and precise control over material properties. Common methods include:
Lithography
Lithography techniques, such as electron-beam lithography and photolithography, are used to pattern quantum wires on semiconductor substrates. These methods allow for precise control over the dimensions and placement of the wires.
Molecular Beam Epitaxy (MBE)
MBE is a method for growing crystalline layers to fabricate quantum wires. In this technique, beams of atoms or molecules are directed at a heated substrate, where they condense and form thin films with atomic precision.
Chemical Vapor Deposition (CVD)
CVD involves the chemical reaction of gaseous precursors on a substrate to form solid materials. This technique is often used to grow carbon-based quantum wires, such as carbon nanotubes.
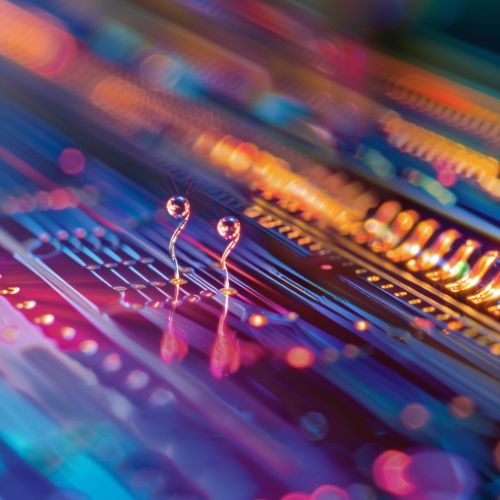
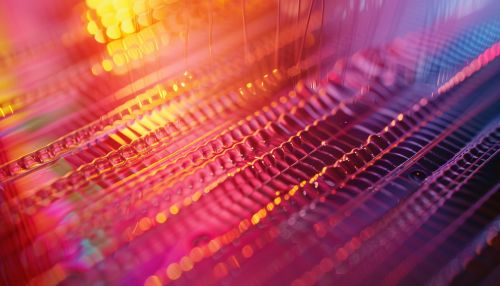
Electronic Properties
Quantum wires exhibit unique electronic properties due to the quantization of energy levels. These properties include:
Conductance Quantization
In quantum wires, conductance is quantized in units of \(2e^2/h\), where \(e\) is the electron charge and \(h\) is Planck's constant. This phenomenon arises from the discrete nature of the electronic states in the wire.
Ballistic Transport
Electrons can travel through quantum wires with minimal scattering, a regime known as ballistic transport. This occurs when the length of the wire is shorter than the electron mean free path, allowing electrons to move without resistance.
Coulomb Blockade
In narrow quantum wires, electron-electron interactions can lead to the Coulomb blockade effect, where the addition of a single electron requires a significant amount of energy, effectively preventing further electron flow at low temperatures.
Optical Properties
The optical properties of quantum wires are also influenced by their reduced dimensionality:
Quantum Confinement
Quantum confinement leads to discrete energy levels, which can result in sharp optical absorption and emission spectra. This property is useful in applications such as quantum dot lasers and single-photon sources.
Excitonic Effects
Excitons, which are bound states of electrons and holes, are more strongly bound in quantum wires due to the enhanced Coulomb interaction in one dimension. This results in higher exciton binding energies and altered optical transitions.
Thermal Properties
The thermal properties of quantum wires differ significantly from bulk materials:
Phonon Confinement
The confinement of phonons, or lattice vibrations, in quantum wires leads to changes in thermal conductivity. This can result in reduced thermal conductivity, which is beneficial for thermoelectric applications.
Enhanced Thermoelectric Efficiency
Quantum wires can exhibit enhanced thermoelectric efficiency due to the increased density of states at the Fermi level and reduced thermal conductivity. This makes them promising candidates for thermoelectric devices.
Applications
Quantum wires have a wide range of potential applications due to their unique properties:
Nanoelectronics
Quantum wires are used in nanoelectronic devices, such as transistors and interconnects, where their small size and high conductance are advantageous.
Photonics
In photonics, quantum wires are used in devices like lasers and light-emitting diodes (LEDs) due to their sharp optical transitions and high efficiency.
Quantum Computing
Quantum wires are explored for use in quantum computing as they can serve as qubits or interconnects in quantum circuits.
Challenges and Future Directions
Despite their potential, several challenges remain in the development and application of quantum wires:
Fabrication Precision
Achieving precise control over the dimensions and material properties of quantum wires remains a significant challenge. Advances in fabrication techniques are needed to improve reproducibility and scalability.
Integration with Existing Technologies
Integrating quantum wires with existing semiconductor technologies requires overcoming compatibility issues and developing new fabrication processes.
Understanding Fundamental Properties
Further research is needed to fully understand the fundamental properties of quantum wires, including their electronic, optical, and thermal behaviors.