The Physics of Laser Cooling and Trapping of Atoms
Introduction
Laser cooling and atom trapping are two interconnected techniques used in the field of atomic physics to manipulate the behavior of atoms. These methods exploit the properties of light and matter to reduce the kinetic energy of atoms, effectively cooling them to temperatures near absolute zero. This article delves into the underlying physics of these techniques, exploring the principles and mechanisms that make laser cooling and atom trapping possible.
Principles of Laser Cooling
Laser cooling is a technique that utilizes the interaction between light and matter to reduce the kinetic energy of atoms or molecules. This process is based on the principles of quantum mechanics, specifically the Doppler effect and the momentum conservation law.
When an atom absorbs a photon from a laser beam, it experiences a change in momentum equal to the momentum of the photon. If the atom is moving towards the laser source, it will absorb more photons due to the Doppler effect, which increases the frequency of the light from the perspective of the atom. This leads to a net force opposing the atom's motion, effectively slowing it down and reducing its kinetic energy. This process is known as Doppler cooling, one of the most common forms of laser cooling.
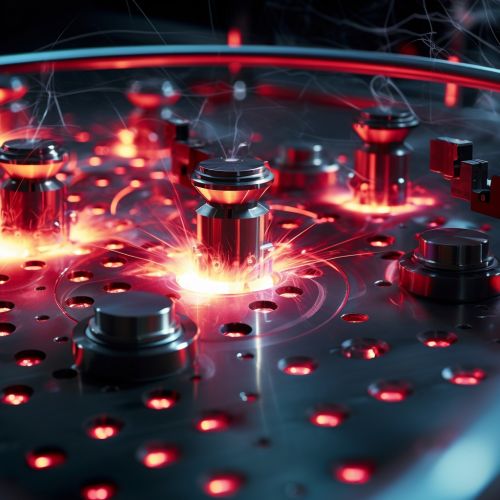
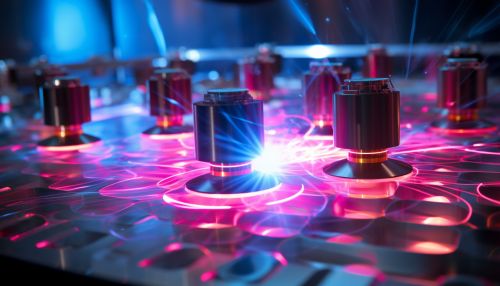
Techniques of Laser Cooling
Several techniques have been developed to implement laser cooling, each with its unique characteristics and applications. These include Doppler cooling, Sisyphus cooling, Resolved sideband cooling, and Optical molasses.
Doppler cooling is the simplest and most commonly used technique. It uses a pair of counter-propagating laser beams to cool atoms. The lasers are tuned slightly below the atomic resonance frequency, causing atoms moving towards each beam to preferentially absorb photons and slow down.
Sisyphus cooling, on the other hand, involves the use of polarized light and a magnetic field to create a potential energy landscape for the atoms. The atoms climb this "hill" and lose kinetic energy in the process, effectively cooling them down.
Resolved sideband cooling is a technique used to cool ions trapped in a Paul trap or a Penning trap. It involves the use of a tightly focused laser beam to selectively excite the ions' motion, causing them to lose energy and cool down.
Optical molasses is a technique that combines multiple laser beams to create a three-dimensional cooling environment for the atoms. The lasers are detuned below the atomic resonance frequency, creating a viscous medium that slows down the atoms, hence the name "optical molasses".
Principles of Atom Trapping
Atom trapping is a technique used to confine atoms in a small region of space. This is achieved by creating a potential well using electromagnetic fields, where the atoms are attracted towards the minimum of the potential. The most common types of atom traps are Magnetic traps, Optical traps, and Magneto-optical traps.
Magnetic traps use magnetic fields to create the potential well. Atoms with a magnetic moment are attracted towards regions of low magnetic field, effectively trapping them.
Optical traps, also known as optical tweezers, use focused laser beams to trap atoms. The atoms are attracted towards the high-intensity region of the laser beam, effectively trapping them.
Magneto-optical traps combine the principles of laser cooling and magnetic trapping to confine and cool atoms simultaneously. They use a combination of laser beams and a magnetic field to create a potential well and cool the atoms.
Techniques of Atom Trapping
The techniques used for atom trapping largely depend on the type of trap being used. In a magnetic trap, a pair of coils in a Helmholtz configuration is often used to create a uniform magnetic field. The atoms are then cooled and trapped in the region of low magnetic field.
In an optical trap, a tightly focused laser beam is used to trap the atoms. The laser beam is tuned to a frequency below the atomic resonance frequency, creating a potential well that attracts the atoms.
In a magneto-optical trap, six laser beams and a pair of Helmholtz coils are used. The laser beams are detuned below the atomic resonance frequency and oriented in three orthogonal directions. The Helmholtz coils create a magnetic field gradient that confines the atoms.
Applications of Laser Cooling and Atom Trapping
The ability to cool and trap atoms has opened up a wide range of applications in various fields. These include precision measurements, quantum computing, atomic clocks, and Bose-Einstein condensation.
Precision measurements, such as the measurement of the fine-structure constant and the gravitational constant, have been improved significantly with the use of laser-cooled and trapped atoms. The high degree of control over the atomic motion allows for more accurate and precise measurements.
Quantum computing is another field that benefits greatly from laser cooling and atom trapping. Quantum bits, or qubits, can be implemented using trapped ions, which can be manipulated with lasers to perform quantum computations.
Atomic clocks, which are the most accurate timekeeping devices, also rely on laser cooling and atom trapping. The cesium atomic clock, for example, uses a fountain of laser-cooled cesium atoms to measure time with extreme precision.
Bose-Einstein condensation, a state of matter where atoms behave as a single quantum entity, was first observed in a gas of laser-cooled and trapped rubidium atoms. This discovery, which earned the 2001 Nobel Prize in Physics, has paved the way for the study of quantum phenomena on a macroscopic scale.
Conclusion
The physics of laser cooling and atom trapping is a fascinating field that combines principles from quantum mechanics, optics, and atomic physics. The ability to manipulate atoms with light and magnetic fields has opened up new avenues of research and led to numerous technological advancements. As our understanding of these techniques continues to grow, so too will their potential applications.