Laser cooling
Introduction
Laser cooling is a technique used to reduce the thermal motion of particles, such as atoms or ions, by using laser light. The process involves the absorption of photons by the particles, which subsequently re-emit them in a random direction. This exchange of momentum between the particles and the photons results in a net cooling effect, effectively reducing the kinetic energy of the particles.
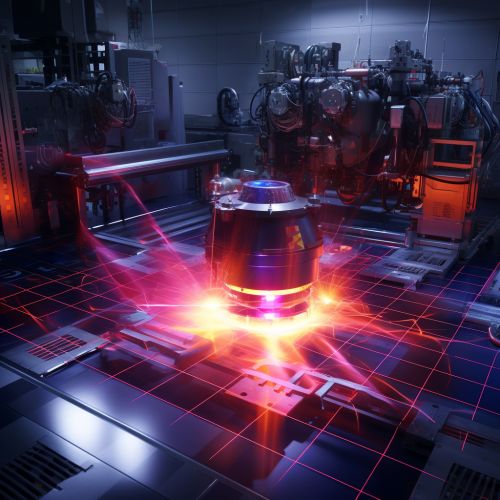
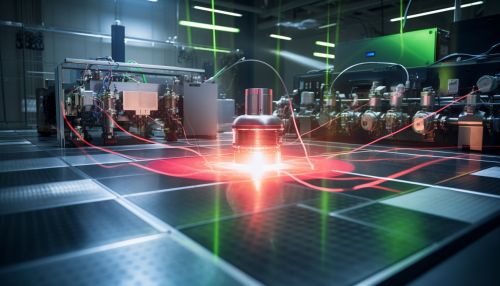
Principle of Operation
The fundamental principle behind laser cooling is the Doppler effect, which is the change in frequency or wavelength of a wave in relation to an observer moving relative to the wave source. When a particle moving towards a laser source absorbs a photon, it experiences a momentum change and slows down. As the particle re-emits the photon in a random direction, the net effect is a reduction in the particle's velocity, effectively cooling it.
Techniques
There are several techniques of laser cooling, each with its own set of advantages and drawbacks. These include Doppler cooling, sub-Doppler cooling techniques such as polarization gradient cooling and Sisyphus cooling, and sub-recoil cooling techniques like Raman sideband cooling and velocity-selective coherent population trapping.
Doppler Cooling
Doppler cooling is the most basic form of laser cooling and is often the first step in the cooling process. It utilizes the Doppler effect to cool particles to temperatures on the order of hundreds of microkelvin. However, due to the limitations imposed by the Doppler cooling limit, also known as the recoil limit, other techniques are required to achieve lower temperatures.
Sub-Doppler Cooling
Sub-Doppler cooling techniques, including polarization gradient cooling and Sisyphus cooling, are used to cool particles below the Doppler cooling limit. These techniques exploit the polarization properties of light and the quantum mechanical nature of the particles to achieve cooling to temperatures on the order of tens of microkelvin.
Sub-Recoil Cooling
Sub-recoil cooling techniques, such as Raman sideband cooling and velocity-selective coherent population trapping, are used to cool particles to temperatures below the recoil limit. These techniques make use of the quantum mechanical properties of the particles and the interaction between light and matter to achieve cooling to temperatures on the order of nanokelvin.
Applications
Laser cooling has a wide range of applications in various fields of science and technology. These include precision measurement, quantum information processing, atomic clocks, and Bose-Einstein condensation.
Precision Measurement
In precision measurement, laser cooling is used to reduce the thermal motion of particles, thereby increasing the accuracy of measurements. This is particularly useful in experiments that require high precision, such as tests of fundamental physics and measurements of fundamental constants.
Quantum Information Processing
In quantum information processing, laser cooling is used to prepare quantum systems in low-entropy states, which are essential for quantum computing and quantum communication. By reducing the thermal motion of particles, laser cooling allows for the precise manipulation and control of quantum states.
Atomic Clocks
Atomic clocks, which are the most accurate timekeeping devices currently available, rely on laser cooling to reduce the thermal motion of atoms. This increases the accuracy of the clock by reducing the Doppler broadening of the atomic transition frequency.
Bose-Einstein Condensation
In Bose-Einstein condensation, laser cooling is used to cool a dilute gas of bosonic atoms to temperatures very close to absolute zero. At these temperatures, a large fraction of the atoms occupy the lowest quantum state, resulting in the formation of a Bose-Einstein condensate.
Conclusion
Laser cooling is a powerful technique that has revolutionized various fields of science and technology. By reducing the thermal motion of particles, it allows for high-precision measurements, precise manipulation of quantum states, and the exploration of novel quantum phenomena. Despite its successes, there are still many challenges and opportunities for further research and development in this exciting field.