Photonic Bandgap
Introduction
A photonic crystal is a periodic optical nanostructure that affects the motion of photons in much the same way that ionic lattices affect electrons in solids. Photonic crystals occur in nature in the form of structural coloration and animal reflectors, and, in different forms, promise to revolutionize industries such as aviation, telecommunication, and optical computing.
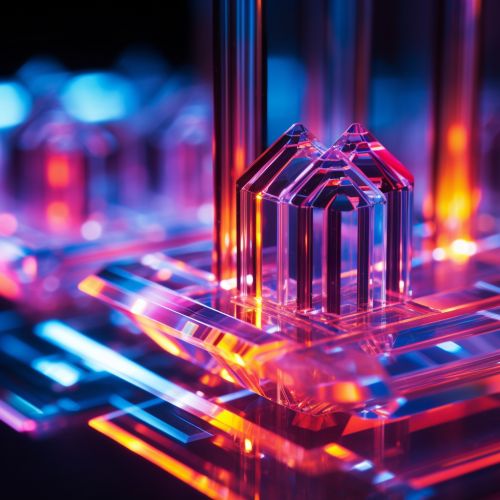
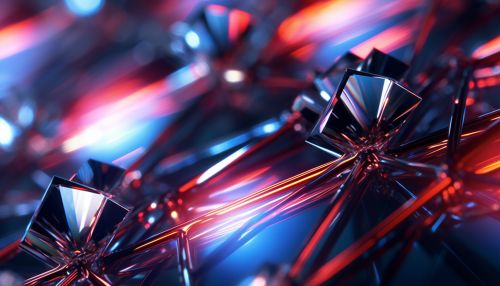
Theory and Principles
The fundamental principle behind photonic crystals is the creation of a photonic bandgap, a range of photon energies that cannot propagate through the crystal. This is analogous to the electronic bandgap found in semiconductors and insulators. The photonic bandgap is created by a periodic structure with a refractive index that varies on a length scale comparable to the wavelength of light. This periodicity can be one-, two-, or three-dimensional.
One-Dimensional Photonic Crystals
One-dimensional photonic crystals, also known as Bragg gratings, consist of alternating layers of materials with different refractive indices. The photonic bandgap in these structures is due to the interference of light waves reflecting off the different layers.
Two-Dimensional Photonic Crystals
Two-dimensional photonic crystals are structures in which the refractive index varies in two dimensions. These can be created by etching holes in a slab of material or by depositing materials in a two-dimensional lattice pattern. The photonic bandgap in these structures is due to the interference of light waves reflecting off the different parts of the lattice.
Three-Dimensional Photonic Crystals
Three-dimensional photonic crystals are the most complex and have the potential to completely control the flow of light. They can be created by stacking two-dimensional photonic crystals in a three-dimensional lattice, or by using techniques such as direct laser writing to create a three-dimensional structure directly.
Applications
Photonic crystals have a wide range of potential applications, from low-power optical computing to high-efficiency solar cells. The ability to control the flow of light at the nanoscale opens up possibilities for new technologies that were previously unattainable.
Optical Computing
In optical computing, photonic crystals can be used to create optical transistors that switch light on and off, analogous to the way electronic transistors switch electrons. This could lead to computers that are faster, more efficient, and generate less heat than current electronic computers.
Telecommunications
In telecommunications, photonic crystals can be used to create filters that only allow certain wavelengths of light to pass through. This could be used to increase the capacity of fiber-optic cables, as different data streams could be sent on different wavelengths of light.
Solar Cells
In solar cells, photonic crystals can be used to trap light and increase the amount of energy that is absorbed. This could lead to more efficient solar cells that can generate more electricity from the same amount of sunlight.
Challenges and Future Research
While photonic crystals hold great promise, there are also significant challenges to overcome. One of the main challenges is fabricating three-dimensional photonic crystals with a large, complete photonic bandgap. Current fabrication techniques are limited and often result in structures with defects or incomplete bandgaps.
Future research in photonic crystals is likely to focus on improving fabrication techniques and exploring new materials. There is also ongoing research into the fundamental physics of photonic crystals, which could lead to new insights and applications.