X-ray Fluorescence
Introduction
X-ray fluorescence (XRF) is a non-destructive analytical technique used to determine the elemental composition of materials. XRF works by striking a sample with an X-ray beam from an X-ray tube, causing the elements within the sample to emit secondary (or fluorescent) X-rays of characteristic energies that are unique to each element.
Principle of Operation
The principle of operation of XRF hinges on the excitation and subsequent relaxation of atoms in a sample. When an atom in the sample is struck by a high-energy X-ray photon, an electron in one of the atom's inner orbital shells is ejected. This creates an electron vacancy that is filled by an electron from an outer orbital shell. The energy difference between the inner and outer shells is released in the form of a secondary X-ray photon, which is detected and measured by an X-ray detector. The energy of this secondary X-ray photon is characteristic of the element from which it was emitted, allowing for elemental identification.
X-ray Sources
The X-ray source in an XRF instrument is typically an X-ray tube, which generates X-rays by accelerating electrons onto a metal target. The energy of the X-rays produced by the tube can be controlled by adjusting the voltage and current applied to the tube. This allows for the excitation of a wide range of elements, from light elements such as beryllium to heavy elements like uranium.
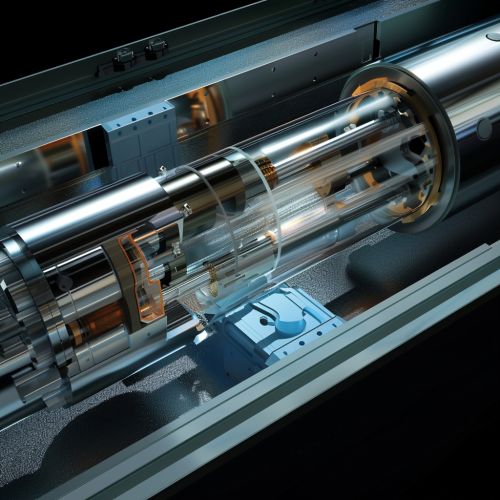
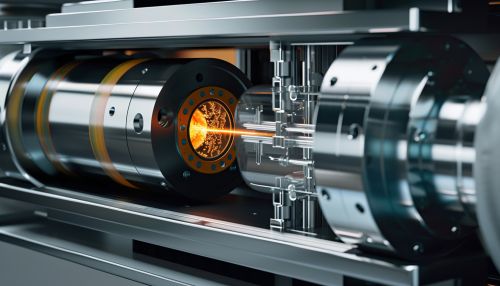
Detectors
XRF detectors are devices that measure the energy of the fluorescent X-rays emitted by the sample. There are two main types of detectors used in XRF: gas-filled detectors and solid-state detectors. Gas-filled detectors, such as proportional counters and Geiger-Mueller counters, operate by ionizing a gas-filled chamber with the incoming X-ray photons. Solid-state detectors, such as silicon drift detectors (SDDs) and high-purity germanium (HPGe) detectors, operate by ionizing a solid semiconductor material. SDDs are currently the most commonly used detectors in XRF due to their superior energy resolution and count rate capabilities.
Sample Preparation
Sample preparation for XRF analysis can vary greatly depending on the type of sample and the specific application. For solid samples, preparation methods can range from simply placing the sample in a sample cup for loose powders or granules, to pressing the sample into a pellet or fusing it into a glass bead for more complex matrices. Liquid samples can be analyzed directly or after drying or digestion, while gaseous samples can be analyzed directly in a sealed cell.
Applications
XRF is used in a wide variety of applications due to its ability to quickly and accurately determine the elemental composition of a sample. Some of the most common applications of XRF include the analysis of metals and alloys, environmental samples, geological samples, and archaeological artifacts. In addition, XRF is also used in the semiconductor industry for thin film analysis, and in the petroleum industry for sulfur analysis in fuels.
Advantages and Limitations
One of the main advantages of XRF is its non-destructive nature, which allows for the analysis of valuable or irreplaceable samples. XRF is also a rapid technique, with most analyses taking only a few minutes. Furthermore, XRF can analyze a wide range of elements, from beryllium to uranium, and can detect concentrations from parts-per-million (ppm) levels to 100%.
However, XRF also has some limitations. For example, it has difficulty detecting light elements (those with atomic numbers less than 11), and its sensitivity decreases for elements with lower atomic numbers. Additionally, XRF is a surface technique, meaning it only analyzes the top layer of the sample, which can be a limitation for samples with layered or complex matrices.