Superfluidity
Introduction
Superfluidity is a state of matter in which matter behaves like a fluid with zero viscosity. This state is achieved at very low temperatures, and the substance moves without losing kinetic energy. The phenomenon was first discovered in liquid helium, by Pyotr Kapitsa and John F. Allen. It is also found in astrophysics, high-energy physics, and theories of quantum gravity.
History
The phenomenon of superfluidity was first discovered in liquid helium by Pyotr Kapitsa and John F. Allen in 1937. They observed that helium II (the low-temperature phase of liquid helium) flows without friction, a behavior now known as superfluidity. This discovery was followed by the theoretical explanation of superfluidity by Lev Landau.
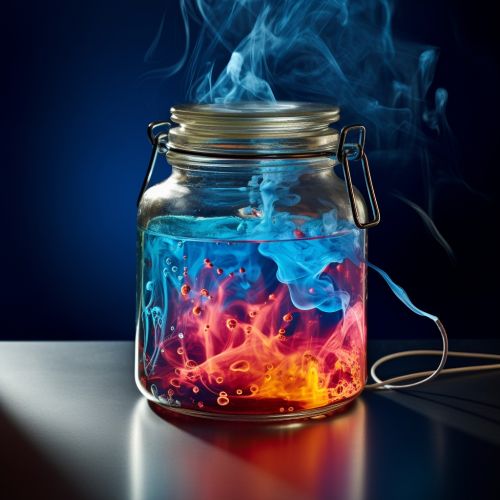
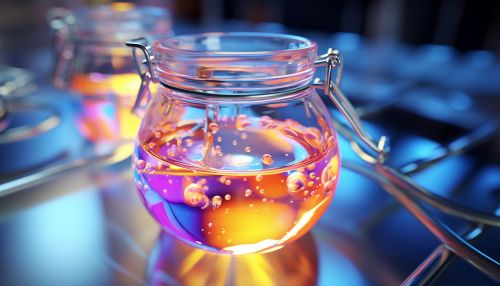
Theoretical Explanation
Superfluidity is a quantum mechanical phenomenon. The theoretical understanding of superfluidity comes from the theory of quantum mechanics, particularly the branch known as quantum field theory. The key idea is that superfluidity is a manifestation of the quantum mechanical principle of Bose-Einstein condensation.
When a system of particles is cooled to temperatures very close to absolute zero, the particles condense into the lowest quantum state, forming a Bose-Einstein condensate. In this state, the particles behave as a single entity, and the system exhibits properties such as superfluidity.
Properties of Superfluids
Superfluids exhibit several unique properties that distinguish them from normal fluids. These properties are a direct result of the quantum mechanical nature of superfluids.
Zero Viscosity
Superfluids have zero viscosity, meaning they can flow without any resistance. This is in contrast to normal fluids, which have a certain amount of viscosity that causes resistance to flow.
Quantum Vortices
In a superfluid, the flow of particles is quantized, leading to the formation of quantum vortices. These vortices are stable, and once formed, they cannot be destroyed.
Thermal Conductivity
Superfluids have extremely high thermal conductivity, much higher than even the best normal conductors. This means that heat can be conducted through a superfluid very efficiently.
Superfluidity in Helium
Superfluidity was first discovered in helium, specifically in the isotope helium-4. When cooled to a temperature below 2.17 Kelvin, helium-4 becomes a superfluid, exhibiting zero viscosity and high thermal conductivity.
Helium-3
Superfluidity has also been observed in the other stable isotope of helium, helium-3. However, the superfluid phase of helium-3 appears at much lower temperatures, below about 0.002 Kelvin.
Superfluidity in Other Systems
While helium is the most well-known example of a superfluid, superfluidity can occur in other systems as well.
Superconductors
In certain materials, the electrons can form pairs (known as Cooper pairs), and these pairs can condense to form a superfluid. This superfluid of electron pairs is what gives rise to superconductivity.
Ultracold Atomic Gases
Superfluidity has been observed in ultracold atomic gases, such as rubidium and lithium. These gases are cooled to temperatures close to absolute zero, and under these conditions, they can form a Bose-Einstein condensate and exhibit superfluidity.
Applications of Superfluidity
Superfluidity has several potential applications in various fields of science and technology.
Cooling Systems
Due to their high thermal conductivity, superfluids can be used in cooling systems. For example, superfluid helium is used to cool the magnets in particle accelerators.
Quantum Computing
The properties of superfluids could be used in the development of quantum computers. In particular, the ability of superfluids to form stable quantum vortices could be used to store and manipulate quantum information.
Astrophysics
Superfluidity is believed to occur in the cores of neutron stars, and this could have important implications for our understanding of these exotic objects.
See Also
References
1. P. Kapitza, "Viscosity of Liquid Helium below the λ-Point", Nature 141, 74 (1938). 2. J. F. Allen and A. D. Misener, "Flow of Liquid Helium II", Nature 141, 75 (1938). 3. L. D. Landau, "Theory of the Superfluidity of Helium II", Physical Review 60, 356 (1941).