Super-Resolution Microscopy
Introduction
Super-resolution microscopy is a form of light microscopy that surpasses the diffraction limit of standard microscopy. This technique allows for the observation of structures and processes at a molecular level, providing a greater understanding of biological systems and their functions. Super-resolution microscopy has revolutionized the field of cell biology, enabling researchers to study cellular structures and processes with unprecedented detail.
History
The concept of super-resolution microscopy was first introduced in the late 20th century, as a response to the limitations of traditional optical microscopy. The diffraction limit, defined by the Abbe limit, restricts the resolution of an optical microscope to approximately half the wavelength of the light used. This limit was first described by Ernst Abbe in 1873 and has been a fundamental constraint in light microscopy ever since.
The development of super-resolution techniques has been driven by the need to visualize structures and processes at the nanoscale. The first super-resolution technique, Near-Field Scanning Optical Microscopy (NSOM), was developed in the 1980s. This was followed by the development of various other techniques, such as Stimulated Emission Depletion (STED) microscopy, Structured Illumination Microscopy (SIM), and Stochastic Optical Reconstruction Microscopy (STORM).
Principles
Super-resolution microscopy techniques overcome the diffraction limit by exploiting various properties of light and matter. These techniques can be broadly divided into deterministic super-resolution techniques and stochastic super-resolution techniques.
Deterministic super-resolution techniques, such as STED and SIM, use specific patterns of light to either suppress or enhance the fluorescence of certain molecules. This allows for the selective illumination of a subset of molecules, thereby increasing the resolution.
Stochastic super-resolution techniques, such as STORM and Photoactivated Localization Microscopy (PALM), rely on the random switching of fluorescence in individual molecules. By localizing the position of each molecule when it is in the fluorescent state, a high-resolution image can be constructed.
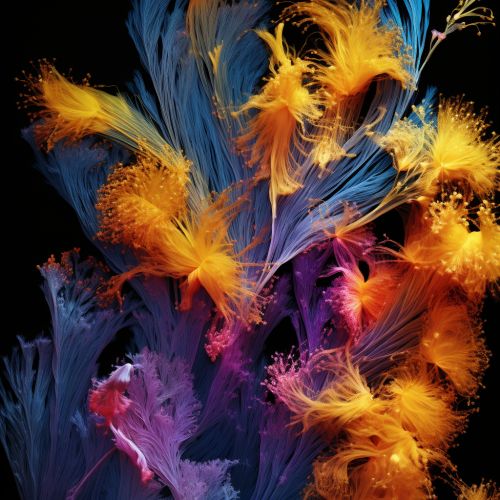
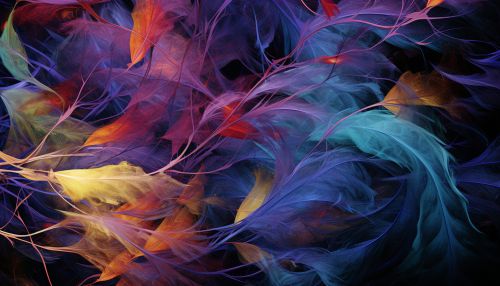
Techniques
There are several super-resolution microscopy techniques, each with its own advantages and disadvantages. The choice of technique depends on the specific requirements of the experiment, such as the desired resolution, the type of sample, and the available resources.
Stimulated Emission Depletion (STED) Microscopy
STED microscopy is a deterministic super-resolution technique that uses two laser beams: an excitation beam and a depletion beam. The excitation beam is used to excite the fluorophores in the sample, while the depletion beam is used to deplete the fluorescence of all fluorophores except those in the center of the excitation beam. This results in a smaller effective point spread function (PSF), thereby increasing the resolution.
Structured Illumination Microscopy (SIM)
SIM is another deterministic super-resolution technique that uses a patterned illumination to enhance the spatial frequencies in the image. This results in a higher resolution image. SIM can be used with a wide range of fluorophores and is less damaging to the sample compared to other super-resolution techniques.
Stochastic Optical Reconstruction Microscopy (STORM)
STORM is a stochastic super-resolution technique that relies on the random switching of fluorescence in individual molecules. By localizing the position of each molecule when it is in the fluorescent state, a high-resolution image can be constructed. STORM provides a high resolution and is compatible with a wide range of fluorophores.
Photoactivated Localization Microscopy (PALM)
PALM is similar to STORM, but uses photoactivatable fluorophores. These fluorophores can be switched from a non-fluorescent state to a fluorescent state using light of a specific wavelength. By localizing the position of each molecule when it is in the fluorescent state, a high-resolution image can be constructed.
Applications
Super-resolution microscopy has a wide range of applications in biological and medical research. It is used to study cellular structures, such as the cytoskeleton, organelles, and membranes, at a molecular level. It is also used to study biological processes, such as protein-protein interactions, signal transduction, and cell division.
In addition to biological research, super-resolution microscopy is also used in materials science to study the structure and properties of materials at the nanoscale.
Limitations and Challenges
Despite its advantages, super-resolution microscopy also has several limitations and challenges. The techniques require specialized equipment and expertise, making them less accessible to many researchers. They also require the use of specific fluorophores, which may not be compatible with all samples.
Furthermore, super-resolution microscopy techniques are limited by the photostability and brightness of the fluorophores. The techniques also have a limited depth of penetration, making them less suitable for imaging thick samples.
Future Directions
The field of super-resolution microscopy is continuously evolving, with new techniques and improvements being developed. Future directions include the development of new fluorophores with improved properties, the integration of super-resolution microscopy with other imaging techniques, and the development of computational methods for image analysis and reconstruction.