Stokes Shift
Introduction
The Stokes shift, named after Irish physicist George G. Stokes, is a phenomenon in the field of fluorescence spectroscopy. It is defined as the difference in energy, or wavelength, between positions of the band maxima of the absorption and emission spectra of the same electronic transition. The Stokes shift can be expressed in terms of wavelength, frequency, or energy and is always a positive quantity.
Physical Explanation
The Stokes shift occurs due to the relaxation of the molecular system after excitation. When a molecule absorbs a photon, it is excited from the ground state to a higher energy level. This excited state is often a vibrational level of an electronic state. The molecule then relaxes to the lowest vibrational level of the excited state through non-radiative processes such as internal conversion or vibrational relaxation. This process results in a loss of energy, which is observed as a shift of the emission spectrum to longer wavelengths, or lower energies, compared to the absorption spectrum.
Factors Influencing Stokes Shift
Several factors can influence the magnitude of the Stokes shift. These include the molecular structure, the nature of the electronic transition, the solvent environment, and temperature.
Molecular Structure
The Stokes shift is sensitive to changes in molecular structure during the electronic transition. For example, in molecules with flexible structures, the excited state may lead to a significant change in molecular geometry, resulting in a large Stokes shift.
Nature of the Electronic Transition
The nature of the electronic transition also plays a role in the Stokes shift. Transitions involving charge transfer or the creation of an excited state dipole can result in significant changes in the electron distribution, leading to large Stokes shifts.
Solvent Environment
The solvent environment can also influence the Stokes shift. Solvent molecules can interact with the excited molecule, stabilizing certain electronic states and thus affecting the energy difference between the absorption and emission maxima. Polar solvents, in particular, can significantly increase the Stokes shift.
Temperature
Temperature can also affect the Stokes shift. Higher temperatures increase the population of higher vibrational levels in the ground state, which can lead to a decrease in the Stokes shift.
Measurement of Stokes Shift
The Stokes shift is typically measured using fluorescence spectroscopy. The absorption and emission spectra are recorded, and the wavelengths or energies of the maxima are determined. The Stokes shift is then calculated as the difference between these two values.
Applications of Stokes Shift
The Stokes shift has several important applications in various fields such as analytical chemistry, biochemistry, and materials science.
Analytical Chemistry
In analytical chemistry, the Stokes shift is used in the design of fluorescence-based sensors and assays. The large Stokes shift allows for the separation of the excitation and emission wavelengths, reducing the interference from scattered excitation light and improving the sensitivity of the measurement.
Biochemistry
In biochemistry, the Stokes shift is used in the study of protein structure and dynamics. Changes in the Stokes shift can provide information about the local environment of the fluorescent probe, such as changes in polarity or hydrogen bonding.
Materials Science
In materials science, the Stokes shift is used in the design of luminescent materials, such as organic light-emitting diodes (OLEDs) and quantum dots. Materials with large Stokes shifts are desirable as they can help to reduce self-absorption and improve the efficiency of the device.
See Also
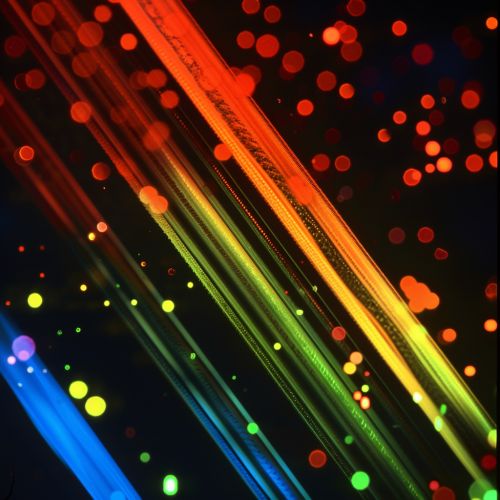
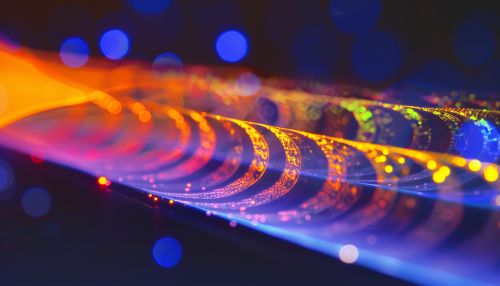