Splice site
Introduction
A splice site is a specific sequence within a pre-mRNA (precursor messenger RNA) transcript that is recognized and utilized by the splicing machinery during the process of RNA splicing. This process is essential for the maturation of pre-mRNA into functional mRNA, which can then be translated into proteins. Splice sites are critical for the accurate removal of introns (non-coding regions) and the joining of exons (coding regions) in eukaryotic genes.
Structure of Splice Sites
Splice sites are composed of several key elements that are recognized by the splicing machinery. These elements include the 5' splice site (donor site), the 3' splice site (acceptor site), the branch point, and the polypyrimidine tract.
5' Splice Site
The 5' splice site is located at the junction between the end of an exon and the beginning of an intron. It typically contains a conserved GU dinucleotide at the 5' end of the intron. The consensus sequence for the 5' splice site is AG|GURAGU, where "|" indicates the exon-intron boundary and "R" represents a purine (adenine or guanine).
3' Splice Site
The 3' splice site is found at the junction between the end of an intron and the beginning of an exon. It usually contains a conserved AG dinucleotide at the 3' end of the intron. The consensus sequence for the 3' splice site is YAG|, where "Y" represents a pyrimidine (cytosine or uracil).
Branch Point
The branch point is a conserved adenine nucleotide located upstream of the 3' splice site. It plays a crucial role in the formation of the lariat structure during splicing. The branch point is typically found within a consensus sequence of YNYURAC, where "N" represents any nucleotide.
Polypyrimidine Tract
The polypyrimidine tract is a sequence rich in pyrimidines (cytosine and uracil) located between the branch point and the 3' splice site. This region enhances the recognition of the 3' splice site by the splicing machinery.
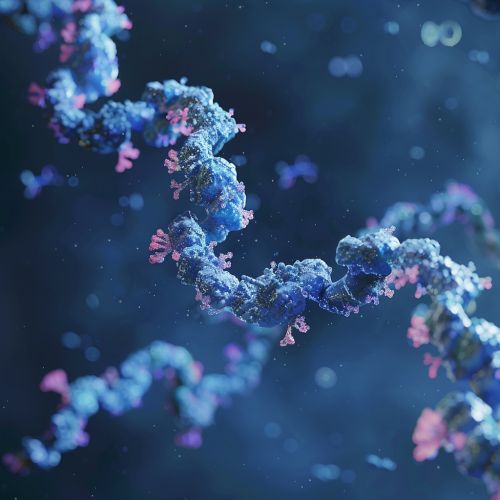
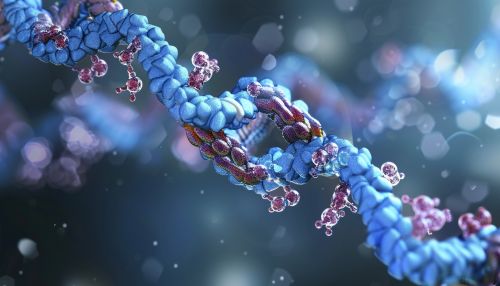
Splicing Mechanism
The splicing process is carried out by a large ribonucleoprotein complex known as the spliceosome. The spliceosome is composed of five small nuclear ribonucleoproteins (snRNPs) and numerous associated proteins.
Assembly of the Spliceosome
The splicing process begins with the recognition of the 5' splice site by the U1 snRNP. This is followed by the binding of the U2 snRNP to the branch point. The U4/U6 and U5 snRNPs then join the complex, forming the mature spliceosome.
Catalysis of Splicing
The spliceosome undergoes a series of conformational changes that bring the 5' splice site, branch point, and 3' splice site into close proximity. This allows for the catalysis of two transesterification reactions. In the first reaction, the 2' hydroxyl group of the branch point adenine attacks the 5' splice site, forming a lariat structure. In the second reaction, the 3' hydroxyl group of the upstream exon attacks the 3' splice site, resulting in the ligation of the exons and the release of the intron lariat.
Alternative Splicing
Alternative splicing is a process by which different combinations of exons are joined together to produce multiple mRNA isoforms from a single gene. This increases the diversity of the proteome and allows for the regulation of gene expression in a tissue-specific or developmental stage-specific manner.
Types of Alternative Splicing
There are several types of alternative splicing, including exon skipping, mutually exclusive exons, alternative 5' splice sites, alternative 3' splice sites, and intron retention.
Regulation of Alternative Splicing
Alternative splicing is regulated by a variety of factors, including splicing enhancers and silencers, which are sequences within the pre-mRNA that promote or inhibit the use of specific splice sites. Additionally, RNA-binding proteins and other regulatory proteins play a crucial role in modulating splicing decisions.
Splicing-Related Diseases
Mutations in splice sites or splicing regulatory elements can lead to aberrant splicing and are associated with a variety of human diseases, including cancer, neurodegenerative disorders, and genetic diseases.
Cancer
Aberrant splicing is commonly observed in cancer and can result in the production of oncogenic protein isoforms or the loss of tumor suppressor functions. For example, mutations in the splice sites of the TP53 gene can lead to the production of dysfunctional p53 protein, contributing to tumorigenesis.
Neurodegenerative Disorders
Splicing defects are also implicated in neurodegenerative disorders such as ALS and SMA. In SMA, mutations in the SMN1 gene affect the splicing of the SMN2 gene, leading to reduced levels of functional SMN protein.
Genetic Diseases
Many genetic diseases are caused by mutations that affect splicing. For example, mutations in the splice sites of the CFTR gene are responsible for certain forms of cystic fibrosis. These mutations result in the production of non-functional CFTR protein, leading to the characteristic symptoms of the disease.
Research and Therapeutic Approaches
Research into the mechanisms of splicing and the development of therapeutic approaches to correct splicing defects is an active area of investigation.
Splice-Switching Oligonucleotides
Splice-switching oligonucleotides (SSOs) are short synthetic RNA molecules that can modulate splicing by binding to specific sequences within the pre-mRNA. SSOs have shown promise in the treatment of diseases such as SMA and DMD.
Small Molecule Modulators
Small molecule modulators of splicing are another therapeutic approach. These compounds can influence the activity of the splicing machinery or the binding of regulatory proteins to pre-mRNA. For example, the small molecule risdiplam has been approved for the treatment of SMA.
Gene Editing
Gene editing technologies, such as CRISPR-Cas9, offer the potential to correct splicing mutations at the genomic level. This approach is being explored for the treatment of various genetic diseases.