Spin Flip
Introduction
In the realm of quantum mechanics and condensed matter physics, the term "spin flip" refers to the process by which the spin of a particle, such as an electron, changes its orientation. This phenomenon is critical in various physical processes and applications, including magnetic resonance imaging (MRI), quantum computing, and the study of magnetic materials. The spin flip is a fundamental concept that bridges the microscopic quantum world with macroscopic physical properties.
Quantum Mechanics and Spin
In quantum mechanics, particles such as electrons possess an intrinsic form of angular momentum known as spin. The spin of an electron can be thought of as a tiny magnetic dipole, which can align in different directions relative to an external magnetic field. The two primary orientations are commonly referred to as "spin-up" and "spin-down." These orientations correspond to the eigenstates of the spin operator along a chosen axis, typically the z-axis.
A spin flip occurs when an electron transitions from one spin state to another. This transition can be induced by various interactions, such as the absorption or emission of a photon, or through interactions with other particles or fields. The probability and dynamics of spin flips are governed by the principles of quantum mechanics, including the Pauli exclusion principle, which states that no two electrons can occupy the same quantum state simultaneously.
Mechanisms of Spin Flip
Magnetic Resonance
One of the most well-known mechanisms for inducing spin flips is magnetic resonance. In electron paramagnetic resonance (EPR) and nuclear magnetic resonance (NMR), an external magnetic field is applied to a sample, causing the magnetic moments of the particles to align with or against the field. When an oscillating magnetic field, typically in the radiofrequency range, is applied perpendicular to the static field, it can induce transitions between the spin states. This process is known as resonance because it occurs at a specific frequency determined by the Zeeman effect.
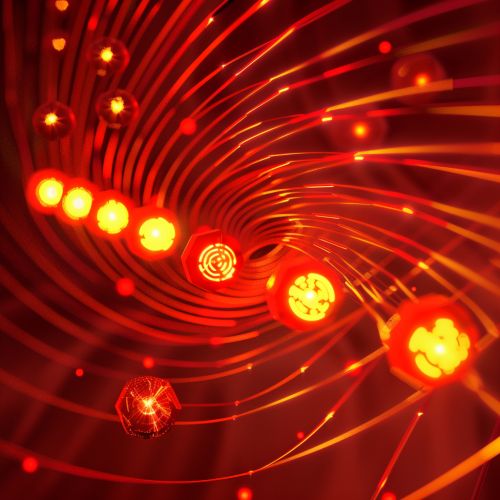
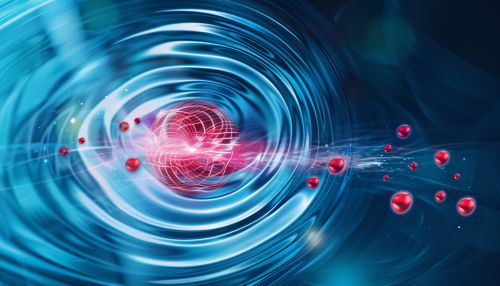
Spin-Orbit Coupling
Spin-orbit coupling is another mechanism that can lead to spin flips. This interaction arises from the coupling between a particle's spin and its orbital motion around a nucleus. In atoms with high atomic numbers, the spin-orbit coupling is significant and can lead to energy level splitting, known as fine structure. Transitions between these levels can involve spin flips, especially when the system is perturbed by external fields or collisions.
Exchange Interaction
In magnetic materials, the exchange interaction between neighboring spins can also cause spin flips. This interaction is responsible for the collective magnetic properties of materials, such as ferromagnetism and antiferromagnetism. In a ferromagnetic material, the spins of adjacent atoms tend to align in the same direction, while in an antiferromagnetic material, they align in opposite directions. Thermal fluctuations or external perturbations can induce spin flips, affecting the overall magnetic state of the material.
Applications of Spin Flip
Magnetic Resonance Imaging (MRI)
One of the most significant applications of spin flip is in magnetic resonance imaging (MRI). MRI is a non-invasive imaging technique used in medical diagnostics to visualize the internal structures of the body. The technique relies on the principles of nuclear magnetic resonance, where the nuclei of certain atoms, such as hydrogen, are subjected to a strong magnetic field and radiofrequency pulses. The resulting spin flips and their relaxation times provide detailed information about the tissue composition and structure.
Quantum Computing
In the field of quantum computing, spin flips play a crucial role in the manipulation of quantum bits, or qubits. Qubits can be realized using the spin states of electrons or nuclei, and precise control over spin flips is essential for performing quantum logic operations. Techniques such as spin echo and Rabi oscillation are employed to manipulate and measure the spin states, enabling the implementation of quantum algorithms.
Spintronics
Spintronics, or spin electronics, is an emerging field that exploits the spin of electrons in addition to their charge for information processing. Devices such as spin valves and magnetic tunnel junctions rely on the controlled spin flip of electrons to achieve high-performance memory and logic functions. The ability to manipulate spin flips at the nanoscale is key to the development of next-generation electronic devices with enhanced speed and efficiency.
Theoretical Models
Bloch Equations
The dynamics of spin flips in magnetic resonance can be described by the Bloch equations. These equations model the time evolution of the magnetization vector in the presence of static and oscillating magnetic fields. The Bloch equations take into account relaxation processes, such as T1 relaxation (longitudinal relaxation) and T2 relaxation (transverse relaxation), which describe the return of the spin system to thermal equilibrium.
Heisenberg Model
In the study of magnetic materials, the Heisenberg model is often used to describe the interactions between spins. This model considers the exchange interaction between neighboring spins and can be extended to include anisotropy and external magnetic fields. The Heisenberg model provides a framework for understanding spin flip dynamics in complex magnetic systems, including the formation of spin waves and magnons.
Experimental Techniques
Electron Spin Resonance (ESR)
Electron spin resonance (ESR), also known as electron paramagnetic resonance (EPR), is a technique used to study materials with unpaired electrons. By applying a magnetic field and sweeping the frequency of an applied microwave field, resonant absorption can be detected, indicating spin flips. ESR is widely used in chemistry, biology, and materials science to investigate the electronic structure and dynamics of paramagnetic species.
Inelastic Neutron Scattering
Inelastic neutron scattering is a powerful technique for probing spin flip excitations in condensed matter systems. Neutrons, due to their magnetic moment, can interact with the spins of atoms in a material. By measuring the energy and momentum transfer during the scattering process, information about spin flip excitations, such as magnons, can be obtained. This technique is essential for studying the magnetic properties of complex materials, including high-temperature superconductors and frustrated magnets.
Challenges and Future Directions
Despite significant advancements, the study of spin flips continues to present challenges. Precise control and measurement of spin states at the quantum level require sophisticated experimental setups and techniques. Additionally, understanding spin flip dynamics in strongly correlated systems and at ultrafast timescales remains an active area of research.
Future directions include the development of novel materials and devices that exploit spin flip phenomena for advanced applications. For instance, the integration of spintronics with traditional semiconductor technology holds promise for the creation of hybrid devices with enhanced functionality. Moreover, the exploration of spin flip processes in low-dimensional systems, such as graphene and topological insulators, offers exciting opportunities for discovering new physical phenomena and applications.