Shock-induced vitrification
Introduction
Shock-induced vitrification is a process where a material transitions into a glassy state due to the application of a high-pressure shock wave. This phenomenon is of significant interest in materials science and condensed matter physics because it provides insights into the behavior of materials under extreme conditions. Vitrification, in general, refers to the transformation of a substance into a glass, a non-crystalline amorphous solid. Shock-induced vitrification specifically involves the rapid application of pressure, leading to unique structural and dynamic properties that differ from those achieved through conventional cooling methods.
Mechanism of Shock-Induced Vitrification
Shock-induced vitrification occurs when a material is subjected to a sudden and intense pressure wave, typically generated by explosive detonation, impact, or high-velocity projectile. The rapid compression and subsequent decompression can force the material into a glassy state. The key factors influencing this process include the magnitude of the shock pressure, the rate of pressure application, and the intrinsic properties of the material.
Pressure and Temperature Conditions
The pressure and temperature conditions during shock-induced vitrification are critical. Typically, pressures range from several gigapascals (GPa) to tens of gigapascals, and temperatures can vary widely depending on the material. The rapid application of pressure can lead to localized heating, which, combined with the high pressure, facilitates the transition to a glassy state.
Atomic and Molecular Dynamics
At the atomic and molecular level, shock-induced vitrification involves complex dynamics. The rapid compression disrupts the crystalline structure, preventing the atoms or molecules from arranging into an ordered lattice. Instead, they become trapped in a disordered, amorphous configuration. The timescale of the shock wave is crucial; it must be short enough to prevent the material from crystallizing but long enough to allow for significant atomic rearrangement.
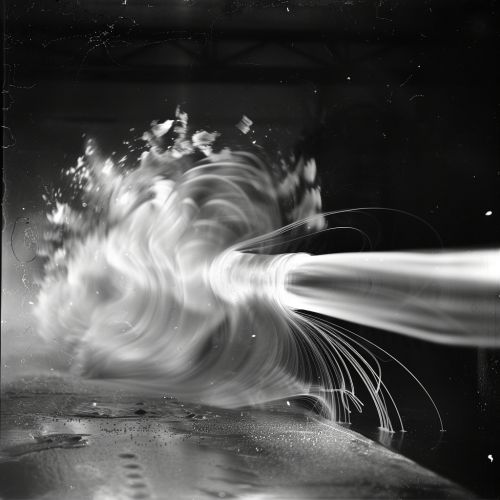
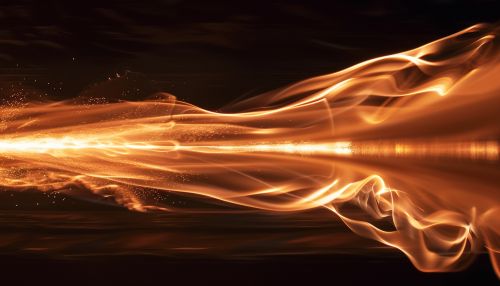
Materials and Applications
Various materials can undergo shock-induced vitrification, including metals, ceramics, polymers, and geological materials. Each material exhibits unique behavior under shock conditions, influenced by its atomic structure, bonding characteristics, and thermal properties.
Metals
Metals such as iron, aluminum, and copper can be vitrified through shock compression. The resulting metallic glasses exhibit high strength, hardness, and resistance to wear and corrosion. These properties make them valuable in applications such as protective coatings, structural components, and magnetic materials.
Ceramics
Ceramics, including silica and alumina, can also undergo shock-induced vitrification. The resulting glassy ceramics are often more resistant to thermal shock and mechanical stress than their crystalline counterparts. Applications include high-temperature insulators, armor materials, and components in aerospace engineering.
Polymers
Polymers can be vitrified through shock compression, leading to materials with enhanced mechanical properties and thermal stability. These shock-vitrified polymers find use in advanced composites, impact-resistant materials, and specialized coatings.
Geological Materials
Shock-induced vitrification is also observed in geological materials, such as quartz and feldspar, during meteorite impacts. The resulting glassy materials, known as impactites, provide valuable information about the conditions and processes during planetary collisions.
Experimental Techniques
Studying shock-induced vitrification requires specialized experimental techniques to generate and analyze the high-pressure conditions.
Shock Wave Generation
Shock waves can be generated using various methods, including explosive detonation, gas guns, and laser-driven shocks. Each method offers different advantages in terms of pressure range, duration, and control.
In-Situ Diagnostics
In-situ diagnostics are essential for monitoring the vitrification process in real-time. Techniques such as X-ray diffraction, Raman spectroscopy, and high-speed photography provide insights into the structural and dynamic changes occurring during shock compression.
Post-Shock Analysis
Post-shock analysis involves examining the vitrified material to determine its properties and structure. Techniques such as transmission electron microscopy (TEM), scanning electron microscopy (SEM), and differential scanning calorimetry (DSC) are commonly used.
Theoretical Models
Theoretical models play a crucial role in understanding shock-induced vitrification. These models help predict the behavior of materials under shock conditions and guide experimental design.
Molecular Dynamics Simulations
Molecular dynamics (MD) simulations are widely used to study the atomic-scale processes during shock-induced vitrification. These simulations provide detailed information about atomic trajectories, bonding changes, and energy dissipation.
Continuum Mechanics Models
Continuum mechanics models describe the macroscopic behavior of materials under shock compression. These models incorporate equations of state, constitutive relations, and thermodynamic principles to predict pressure, temperature, and deformation.
Challenges and Future Directions
Despite significant progress, several challenges remain in the study of shock-induced vitrification.
Experimental Limitations
Generating and controlling shock waves with the desired precision remains challenging. Additionally, real-time diagnostics at extreme conditions require advanced instrumentation and techniques.
Material-Specific Behavior
Different materials exhibit unique responses to shock compression, complicating the development of universal models. Understanding the role of atomic structure, bonding, and defects is crucial for predicting vitrification behavior.
Applications and Scalability
Translating laboratory-scale findings to practical applications requires addressing issues related to scalability, reproducibility, and cost. Developing efficient methods for producing shock-vitrified materials at industrial scales is a key goal.
Conclusion
Shock-induced vitrification is a fascinating area of research with significant implications for materials science and engineering. By understanding the mechanisms, materials, and applications of this process, researchers can develop new materials with enhanced properties for various technological applications. Continued advancements in experimental techniques and theoretical models will further our understanding of this complex phenomenon.