Second-harmonic generation
Introduction
Second-harmonic generation (SHG) is a nonlinear optical process in which two photons with the same frequency interact with a nonlinear material, resulting in the generation of a new photon with twice the frequency of the original photons. This phenomenon is a specific case of frequency doubling, and it plays a crucial role in various applications, including laser technology, optical communications, and material characterization. SHG is a second-order nonlinear process, meaning it only occurs in materials lacking inversion symmetry, such as certain crystals and biological tissues.
Historical Background
The discovery of second-harmonic generation dates back to the early 1960s, following the invention of the laser. The first experimental observation of SHG was reported in 1961 by Peter A. Franken and his colleagues at the University of Michigan. They used a ruby laser to generate the second harmonic in a quartz crystal, marking a significant milestone in the field of nonlinear optics. This discovery opened new avenues for research into the interaction of light with matter and laid the foundation for the development of various nonlinear optical devices.
Theoretical Framework
Nonlinear Optics
Nonlinear optics is the branch of optics that studies the behavior of light in nonlinear media, where the polarization response of the material is not directly proportional to the electric field of the light. In such media, the polarization \( P \) can be expressed as a power series in the electric field \( E \):
\[ P = \varepsilon_0 (\chi^{(1)} E + \chi^{(2)} E^2 + \chi^{(3)} E^3 + \ldots) \]
where \( \varepsilon_0 \) is the permittivity of free space, and \( \chi^{(n)} \) are the nonlinear susceptibilities. The second-order susceptibility \( \chi^{(2)} \) is responsible for second-harmonic generation.
Phase Matching
Phase matching is a critical condition for efficient SHG. It ensures that the generated second-harmonic wave remains in phase with the fundamental wave as they propagate through the nonlinear medium. This condition can be achieved by adjusting the refractive indices of the interacting waves, often through techniques such as birefringence or temperature tuning. The phase-matching condition can be mathematically expressed as:
\[ k_2 = 2k_1 \]
where \( k_1 \) and \( k_2 \) are the wave vectors of the fundamental and second-harmonic waves, respectively.
Materials for Second-Harmonic Generation
Crystals
Certain crystals, such as quartz, lithium niobate, and potassium titanyl phosphate (KTP), are commonly used for SHG due to their high nonlinear coefficients and lack of inversion symmetry. These materials are often engineered to optimize phase matching and maximize conversion efficiency.
Organic Materials
Organic materials, including certain polymers and molecular crystals, have also been explored for SHG applications. These materials offer advantages such as flexibility, low cost, and the ability to be processed into thin films. However, they often exhibit lower nonlinear coefficients compared to inorganic crystals.
Biological Tissues
SHG can also occur in biological tissues, providing a powerful tool for nonlinear optical microscopy. This technique allows for high-resolution imaging of biological structures without the need for external dyes or labels, as SHG is generated intrinsically by certain biological molecules, such as collagen and myosin.
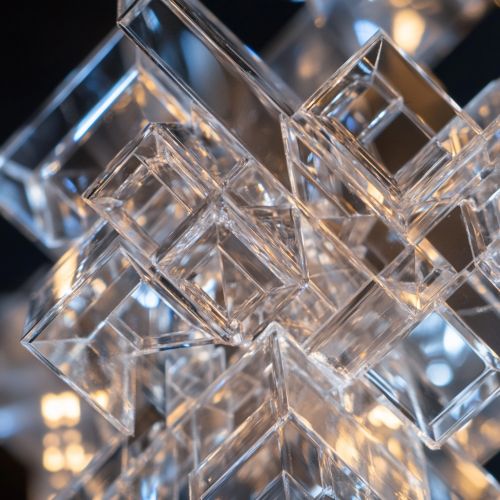
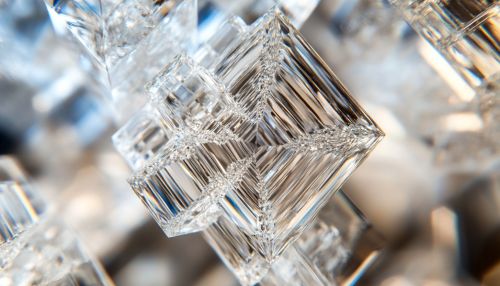
Applications of Second-Harmonic Generation
Laser Technology
In laser technology, SHG is widely used to generate new wavelengths of light. For example, a common application is the conversion of infrared laser light into visible green light using a nonlinear crystal. This process is essential in the development of green laser pointers and other laser-based devices.
Optical Communications
SHG plays a role in optical communications by enabling wavelength conversion and signal processing. It allows for the efficient transfer of information across different frequency bands, enhancing the capacity and flexibility of optical communication networks.
Material Characterization
Second-harmonic generation is a valuable tool for characterizing the properties of materials. By analyzing the SHG response, researchers can gain insights into the symmetry, structure, and electronic properties of various materials. This information is crucial for the development of new materials with tailored optical properties.
Challenges and Future Directions
Despite its widespread applications, SHG faces several challenges, including the need for precise phase matching and the limited availability of suitable nonlinear materials. Ongoing research aims to address these challenges by developing new materials and techniques to enhance SHG efficiency and broaden its applicability.
Future directions in SHG research include the exploration of novel materials, such as metamaterials and nanostructures, which offer unique optical properties and the potential for enhanced nonlinear interactions. Additionally, advances in fabrication techniques may enable the integration of SHG devices into compact, on-chip systems for various technological applications.