Schwinger effect
Overview
The Schwinger effect, named after physicist Julian Schwinger, is a quantum mechanical process where particles are created from a vacuum due to intense electric or magnetic fields. This effect is a direct consequence of the Heisenberg uncertainty principle, which allows for the temporary creation of particle-antiparticle pairs in empty space.
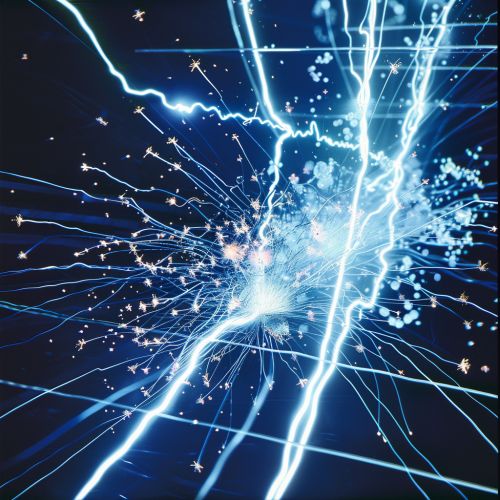
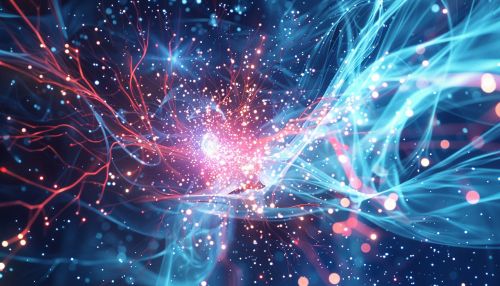
Quantum Field Theory and the Schwinger Effect
In Quantum Field Theory (QFT), the vacuum is not an empty space but a sea of virtual particles that constantly pop in and out of existence. These virtual particles, also known as vacuum fluctuations, are a direct result of the Heisenberg uncertainty principle. The Schwinger effect is a phenomenon where these virtual particles become real particles due to the presence of a strong electric or magnetic field.
The Schwinger effect is a fundamental prediction of QFT and is one of the many examples of how quantum mechanics and classical physics diverge. While classical physics would predict that a vacuum remains empty regardless of the external conditions, QFT predicts that a vacuum can produce particles given the right conditions.
Mathematical Description
The Schwinger effect can be mathematically described using the Dirac equation, a relativistic wave equation derived by British physicist Paul Dirac in 1928. The Dirac equation describes the behavior of fermions, particles with half-integer spin, in the presence of an electromagnetic field.
The probability of pair production per unit volume and per unit time due to the Schwinger effect can be given by the formula:
P = (e^2 E^2 / (4π^2)) * exp(-πm^2c^3 / (ħeE))
where e is the elementary charge, E is the electric field strength, m is the mass of the electron, c is the speed of light, and ħ is the reduced Planck constant.
Experimental Evidence
While the Schwinger effect has not been directly observed in laboratory conditions due to the incredibly high electric fields required, there is indirect evidence for its existence. The most notable evidence comes from the study of Quantum Electrodynamics (QED), the quantum theory of the electromagnetic force.
In QED, the vacuum polarization effect, a process closely related to the Schwinger effect, has been observed. Vacuum polarization involves the creation of electron-positron pairs in the presence of a photon, which is a similar process to the Schwinger effect but involves a different mechanism.
Applications and Implications
The Schwinger effect has important implications in various fields of physics, including Quantum Chromodynamics (QCD), the theory of the strong nuclear force, and Cosmology, the study of the universe's origin, evolution, and eventual fate.
In QCD, the Schwinger effect is related to the confinement of quarks, the fundamental particles that make up protons and neutrons. The strong nuclear force, which binds quarks together, increases with distance, creating a "flux tube" of energy. If the energy in this tube becomes too great, it can cause a Schwinger-like pair production, creating new quarks and antiquarks.
In cosmology, the Schwinger effect is thought to play a role in the early universe's evolution and the production of matter and antimatter. It may also have implications for the study of black holes and the radiation they emit, known as Hawking radiation.