Quantum wire
Introduction
A quantum wire is a one-dimensional (1D) electronic system where the motion of electrons is confined to a single spatial dimension. This confinement leads to unique quantum mechanical effects that are not observed in higher-dimensional systems. Quantum wires are of significant interest in the fields of nanotechnology, quantum computing, and condensed matter physics due to their potential applications and the novel phenomena they exhibit.
Structure and Fabrication
Quantum wires can be fabricated using various methods, including lithography, molecular beam epitaxy, and chemical vapor deposition. These techniques allow for precise control over the dimensions and properties of the wires. The width of a quantum wire is typically on the order of nanometers, which is comparable to the Fermi wavelength of electrons in the material.
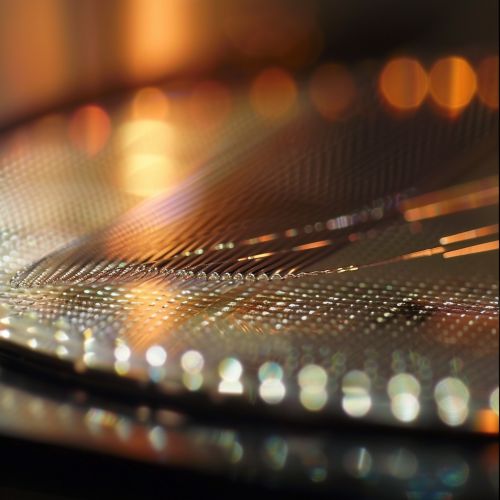
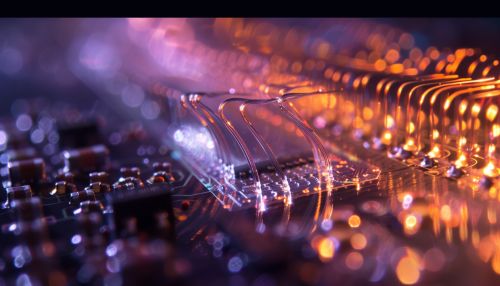
The most common materials used for quantum wires include semiconductors such as silicon, gallium arsenide, and indium phosphide. These materials are chosen for their well-understood electronic properties and compatibility with existing semiconductor technology.
Quantum Confinement and Electronic Properties
In a quantum wire, the electrons are confined in two spatial dimensions, allowing them to move freely only along the length of the wire. This confinement leads to the quantization of energy levels, similar to the particle in a box model in quantum mechanics. The energy levels in a quantum wire are discrete, and the density of states exhibits a step-like structure, in contrast to the continuous density of states in bulk materials.
The electronic properties of quantum wires are significantly influenced by quantum confinement. For instance, the conductance of a quantum wire is quantized in units of 2e^2/h, where e is the elementary charge and h is Planck's constant. This phenomenon, known as quantum conductance, arises due to the formation of discrete transverse modes or subbands in the wire.
Transport Phenomena
The transport properties of quantum wires are governed by quantum mechanical principles. One of the most notable phenomena is ballistic transport, where electrons travel through the wire without scattering. This occurs when the length of the wire is shorter than the electron mean free path. In this regime, the resistance of the wire is independent of its length and is determined solely by the number of available conduction channels.
Another important transport phenomenon in quantum wires is Coulomb blockade, which occurs when the wire is so narrow that the addition of a single electron significantly changes the electrostatic potential. This effect can lead to the formation of Coulomb islands and is crucial for the operation of single-electron transistors.
Interaction Effects
Electron-electron interactions play a significant role in the behavior of quantum wires. These interactions can lead to the formation of Luttinger liquids, a theoretical model describing the collective behavior of interacting electrons in one dimension. Unlike the Fermi liquid theory, which describes electrons in higher dimensions, Luttinger liquid theory predicts that the low-energy excitations in a quantum wire are not quasiparticles but rather collective modes.
The presence of strong electron-electron interactions can also give rise to phenomena such as Wigner crystallization, where electrons arrange themselves in a regular lattice due to their mutual repulsion. This effect is more pronounced in quantum wires with low electron densities.
Spin-Orbit Coupling and Spintronics
Quantum wires are also of interest in the field of spintronics, which exploits the spin degree of freedom of electrons for information processing. In materials with strong spin-orbit coupling, the spin and momentum of electrons are coupled, leading to spin-dependent transport properties. This can result in the formation of spin-polarized currents and the Rashba effect, where an electric field induces a spin splitting of the energy bands.
The ability to control spin transport in quantum wires opens up possibilities for the development of spin-based quantum devices, such as quantum spin Hall effect devices and topological insulators.
Applications
Quantum wires have potential applications in various advanced technologies. In quantum computing, they can be used to create qubits and quantum gates, which are the building blocks of quantum computers. The discrete energy levels and quantized conductance of quantum wires make them suitable for precise control of electronic states, which is essential for quantum information processing.
In the field of nanoelectronics, quantum wires can be used to create ultra-small transistors and interconnects, enabling the development of highly integrated circuits with superior performance. The unique transport properties of quantum wires also make them promising candidates for thermoelectric devices, which convert heat into electrical energy.
Challenges and Future Directions
Despite their potential, there are several challenges associated with the fabrication and integration of quantum wires into practical devices. One of the main challenges is the precise control of the wire dimensions and material properties at the nanoscale. Variations in these parameters can lead to significant changes in the electronic properties of the wires.
Another challenge is the understanding and control of electron-electron interactions and other many-body effects in quantum wires. These interactions can lead to complex behavior that is not yet fully understood and requires further theoretical and experimental investigation.
Future research in the field of quantum wires is likely to focus on the development of new fabrication techniques, the exploration of novel materials, and the investigation of interaction effects. Advances in these areas could lead to the realization of quantum wire-based devices with unprecedented performance and functionality.