Quantum device
Introduction
A quantum device is an apparatus that leverages the principles of quantum mechanics to perform tasks that classical devices cannot achieve efficiently. These devices exploit phenomena such as superposition, entanglement, and quantum tunneling to process information, measure physical quantities, or manipulate matter at the quantum level. Quantum devices are at the forefront of modern physics and engineering, promising revolutionary advancements in computing, communication, sensing, and cryptography.
Fundamental Principles
Superposition
Superposition is a fundamental principle of quantum mechanics where a quantum system can exist in multiple states simultaneously. This principle is starkly different from classical mechanics, where a system can only be in one state at a time. In a quantum device, superposition allows for the representation and processing of a vast amount of information concurrently. For example, a quantum bit or qubit can be in a state of 0, 1, or any quantum superposition of these states, enabling quantum computers to solve complex problems more efficiently than classical computers.
Entanglement
Entanglement is another cornerstone of quantum mechanics, where two or more quantum particles become correlated in such a way that the state of one particle instantaneously influences the state of the other, regardless of the distance separating them. This phenomenon is utilized in quantum devices to create highly secure communication channels and to perform computations that are impossible for classical systems. Entangled qubits in a quantum computer can perform operations on multiple qubits simultaneously, exponentially increasing computational power.
Quantum Tunneling
Quantum tunneling is the phenomenon where particles pass through a potential barrier that they classically shouldn't be able to cross. This effect is harnessed in various quantum devices, such as tunnel diodes and scanning tunneling microscopes, to achieve functionalities that are unattainable with classical devices. Quantum tunneling is also a critical mechanism in quantum computing and quantum cryptography.
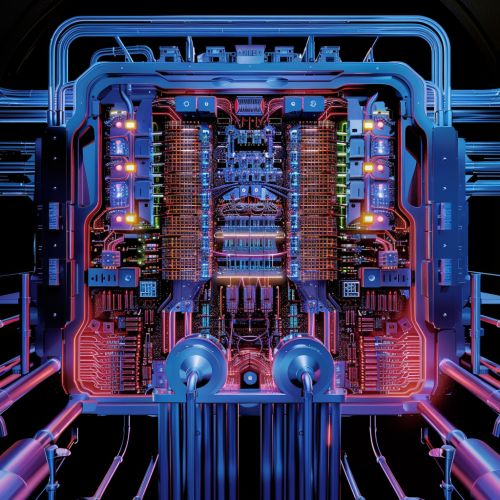
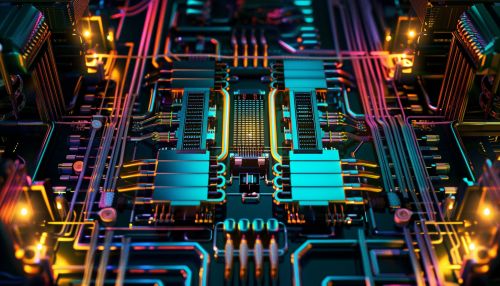
Types of Quantum Devices
Quantum Computers
Quantum computers are perhaps the most well-known quantum devices. They utilize qubits to perform computations that would take classical computers an impractical amount of time to solve. Quantum computers operate using quantum gates and circuits, which manipulate the states of qubits through unitary transformations. These devices hold the potential to revolutionize fields such as cryptography, optimization, and materials science.
Quantum Sensors
Quantum sensors exploit quantum coherence and entanglement to achieve unprecedented sensitivity and precision in measuring physical quantities. Examples include atomic clocks, which are the most accurate timekeeping devices, and magnetometers, which can detect minute changes in magnetic fields. Quantum sensors are crucial in applications ranging from navigation to medical imaging.
Quantum Communication Devices
Quantum communication devices use principles like entanglement and quantum key distribution (QKD) to create secure communication channels. These devices ensure that any attempt to eavesdrop on the communication can be detected, providing a level of security unattainable by classical means. Quantum communication is poised to transform secure data transmission in the digital age.
Applications
Quantum Computing
Quantum computing has the potential to revolutionize various industries by solving problems that are currently intractable for classical computers. Applications include drug discovery, where quantum computers can simulate molecular interactions at an unprecedented level of detail, and cryptography, where they can break classical encryption schemes but also enable new, unbreakable encryption methods.
Quantum Cryptography
Quantum cryptography leverages the principles of quantum mechanics to create secure communication protocols. Quantum key distribution (QKD) is a prominent example, where the security of the key exchange is guaranteed by the laws of quantum mechanics. This technology is critical for protecting sensitive information in an era of increasing cyber threats.
Quantum Sensing
Quantum sensors are used in a variety of fields to achieve measurements with extreme precision. In medicine, quantum sensors can improve imaging techniques, leading to better diagnostics and treatments. In geology, they can detect minute changes in gravitational fields, aiding in the exploration of natural resources.
Challenges and Future Directions
Technical Challenges
Despite their potential, quantum devices face significant technical challenges. Quantum systems are highly susceptible to environmental noise and decoherence, which can disrupt their delicate quantum states. Developing error correction methods and fault-tolerant quantum computing architectures is an ongoing area of research.
Scalability
Scaling up quantum devices to practical, large-scale systems is another major challenge. Current quantum computers are limited in the number of qubits they can effectively manage. Overcoming this limitation requires advances in qubit coherence times, error rates, and inter-qubit connectivity.
Integration with Classical Systems
Integrating quantum devices with existing classical systems is crucial for their practical deployment. This involves developing hybrid architectures that can leverage the strengths of both quantum and classical computing. Research in this area focuses on creating interfaces and protocols that allow seamless interaction between quantum and classical components.
See Also
- Quantum Mechanics
- Quantum Computing
- Quantum Cryptography
- Quantum Sensing
- Quantum Entanglement
- Quantum Superposition