Quantum Repeater
Introduction
A quantum repeater is a device used in quantum communication to extend the distance over which quantum information can be transmitted. Quantum repeaters are essential for the development of large-scale quantum networks, which are necessary for applications such as quantum cryptography, quantum teleportation, and distributed quantum computing. Unlike classical repeaters, which amplify signals to extend their range, quantum repeaters rely on principles of quantum mechanics, such as entanglement and quantum error correction, to preserve the integrity of quantum information over long distances.
Background
Quantum communication exploits the principles of quantum mechanics to transmit information in a fundamentally secure manner. The primary challenge in long-distance quantum communication is the loss and decoherence of quantum states as they travel through optical fibers or free space. Classical repeaters cannot be used to amplify quantum states because the act of measurement collapses the quantum state, destroying the information. Therefore, quantum repeaters are designed to overcome these challenges by using entanglement swapping and quantum error correction techniques.
Principles of Operation
Entanglement Swapping
Entanglement swapping is a key process in the operation of quantum repeaters. It involves creating entangled pairs of particles, such as photons, at different locations and then performing a Bell state measurement on one particle from each pair. This measurement projects the remaining particles into an entangled state, effectively extending the range of entanglement. By repeating this process over multiple segments, entanglement can be distributed over long distances.
Quantum Error Correction
Quantum error correction is another crucial component of quantum repeaters. Quantum states are highly susceptible to errors due to decoherence and noise. Quantum error correction codes, such as the Shor code and the Steane code, are used to detect and correct errors without measuring the quantum state directly. These codes encode quantum information into entangled states of multiple particles, allowing for the recovery of the original information even if some particles are lost or corrupted.
Architecture of Quantum Repeaters
Quantum repeaters typically consist of three main components: quantum memories, entanglement sources, and quantum processors.
Quantum Memories
Quantum memories are devices that can store quantum states for extended periods. They are essential for synchronizing the entanglement swapping process across different segments of the quantum network. Quantum memories are usually based on atomic ensembles, rare-earth ions, or nitrogen-vacancy centers in diamonds.
Entanglement Sources
Entanglement sources generate pairs of entangled particles. These sources can be based on spontaneous parametric down-conversion (SPDC) in nonlinear crystals or four-wave mixing in optical fibers. The quality and rate of entanglement generation are critical factors in the performance of quantum repeaters.
Quantum Processors
Quantum processors perform the necessary operations for entanglement swapping and error correction. They include components such as beam splitters, phase shifters, and single-photon detectors. Quantum processors must operate with high precision and low error rates to ensure the successful transmission of quantum information.
Challenges and Limitations
Despite significant progress in the development of quantum repeaters, several challenges remain.
Decoherence and Noise
Decoherence and noise are major obstacles in the implementation of quantum repeaters. Quantum states are extremely fragile and can be easily disturbed by interactions with the environment. Developing robust quantum memories and error correction techniques is essential to mitigate these effects.
Resource Requirements
Quantum repeaters require a large number of entangled pairs and quantum memories to operate effectively. The resource requirements increase exponentially with the distance, making it challenging to scale up quantum networks.
Technological Limitations
Current technology for generating and detecting single photons, as well as for storing and manipulating quantum states, is still in its infancy. Advances in materials science, photonics, and quantum information theory are needed to overcome these limitations.
Applications
Quantum repeaters have several potential applications in the field of quantum communication and beyond.
Quantum Key Distribution (QKD)
Quantum key distribution is a method for securely exchanging cryptographic keys using quantum mechanics. Quantum repeaters can extend the range of QKD systems, enabling secure communication over continental distances.
Quantum Internet
A quantum internet would connect quantum computers and other quantum devices over long distances, allowing for distributed quantum computing and secure communication. Quantum repeaters are a fundamental building block for the development of a quantum internet.
Fundamental Physics
Quantum repeaters can also be used to test fundamental principles of quantum mechanics, such as Bell's theorem and quantum nonlocality. By enabling long-distance entanglement, quantum repeaters provide a platform for exploring the limits of quantum theory.
Future Directions
Research in quantum repeaters is an active and rapidly evolving field. Several promising directions are being explored to overcome current challenges and improve the performance of quantum repeaters.
Hybrid Quantum Repeaters
Hybrid quantum repeaters combine different physical systems, such as atoms, ions, and photons, to leverage their respective advantages. For example, atoms can serve as long-lived quantum memories, while photons can be used for fast and efficient transmission of quantum information.
Advanced Error Correction
Developing more efficient and robust quantum error correction codes is crucial for the practical implementation of quantum repeaters. New codes, such as topological codes and surface codes, offer promising avenues for improving error correction in quantum networks.
Integrated Photonics
Integrated photonics involves the use of photonic circuits to manipulate and transmit quantum states on a single chip. Advances in integrated photonics could lead to more compact and scalable quantum repeaters, reducing the resource requirements and improving the overall performance.
Conclusion
Quantum repeaters are a critical technology for the realization of long-distance quantum communication and the development of quantum networks. By leveraging principles such as entanglement swapping and quantum error correction, quantum repeaters can extend the range of quantum information transmission while preserving its integrity. Despite the challenges and limitations, ongoing research and technological advancements hold promise for the future of quantum repeaters and their applications in secure communication, distributed computing, and fundamental physics.
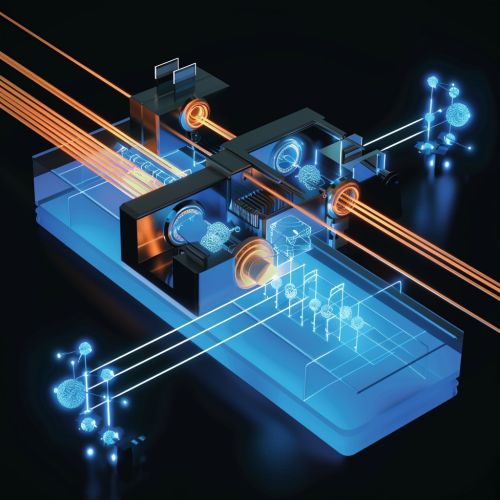
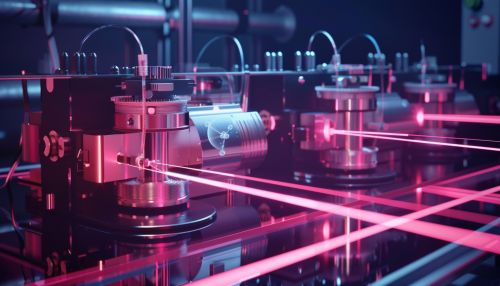
See Also
- Quantum Communication
- Quantum Cryptography
- Quantum Teleportation
- Quantum Internet
- Quantum Error Correction