Quantum Cosmology
Introduction
Quantum cosmology is a branch of theoretical physics that attempts to reconcile the principles of quantum mechanics with those of general relativity, with the goal of understanding the origins and fundamental structure of the universe. This field of study is a subset of cosmology, the scientific study of the universe's large-scale properties and behavior.
Principles of Quantum Cosmology
Quantum cosmology is based on the principles of quantum mechanics, which describe the behavior of particles at the smallest scales, and general relativity, which describes the behavior of matter and energy at the largest scales. These two theories are fundamentally incompatible, leading to paradoxes and inconsistencies when they are applied to the same physical situations. Quantum cosmology seeks to resolve these inconsistencies by developing a quantum theory of gravity, which would provide a unified description of the universe at all scales.
One of the key principles of quantum cosmology is the wave function of the universe, a concept introduced by James Hartle and Stephen Hawking. This wave function is a solution to the Wheeler-DeWitt equation, a fundamental equation in quantum cosmology that combines the principles of quantum mechanics and general relativity. The wave function of the universe describes the probability distribution of the universe's various possible states, providing a quantum mechanical description of the entire universe.
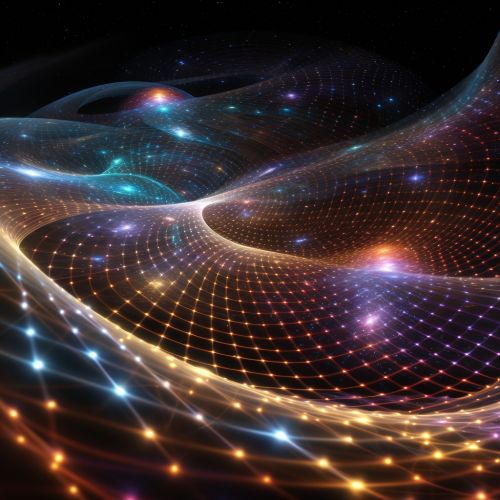
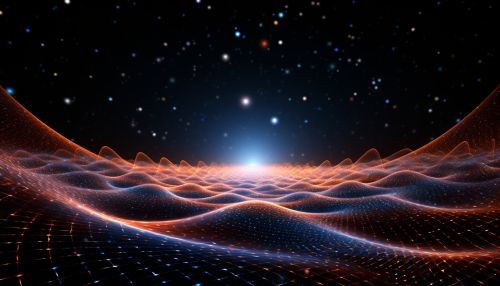
Challenges in Quantum Cosmology
Quantum cosmology faces several significant challenges. One of the most fundamental is the problem of time. In quantum mechanics, time is treated as an external parameter, while in general relativity, it is a dynamical variable that is intertwined with space. This leads to the problem of time in quantum cosmology, which is the question of how to reconcile these two different conceptions of time.
Another major challenge in quantum cosmology is the measurement problem, which is the question of how to interpret the wave function of the universe. In standard quantum mechanics, the wave function is interpreted as a probability distribution for the outcomes of measurements. However, in quantum cosmology, there is no external observer to make measurements, leading to questions about how to interpret the wave function of the universe.
Approaches to Quantum Cosmology
There are several different approaches to quantum cosmology, each with its own strengths and weaknesses. These include the path integral formulation, the canonical quantum gravity approach, and the loop quantum gravity approach.
The path integral formulation, developed by Richard Feynman, involves summing over all possible histories of the universe, each weighted by a phase factor determined by the action of that history. This approach has the advantage of being conceptually simple and mathematically elegant, but it is difficult to apply in practice due to the infinite number of histories that must be summed over.
The canonical quantum gravity approach, developed by Bryce DeWitt and others, involves quantizing the gravitational field in a manner analogous to the quantization of other fields in quantum field theory. This approach has the advantage of being based on well-established principles of quantum mechanics, but it faces the challenge of the problem of time, as mentioned above.
The loop quantum gravity approach, developed by Carlo Rovelli, Lee Smolin, and others, involves quantizing spacetime itself, rather than the gravitational field. This approach has the advantage of providing a potential solution to the problem of time, but it is still a work in progress and has yet to produce a complete theory of quantum gravity.
Implications of Quantum Cosmology
The implications of quantum cosmology are profound and far-reaching. If a successful theory of quantum cosmology can be developed, it would provide a unified description of the universe at all scales, resolving the inconsistencies between quantum mechanics and general relativity.
Such a theory could potentially explain the origins of the universe, including the Big Bang and the subsequent evolution of the universe. It could also provide insights into the nature of dark matter and dark energy, two mysterious components of the universe that are currently not well understood.
In addition, a theory of quantum cosmology could have implications for the multiverse hypothesis, which posits that our universe is just one of many in a vast multiverse. If the wave function of the universe is interpreted as a probability distribution over different universes, then it could provide a scientific basis for the multiverse hypothesis.