Quantum Computing with Quantum Optomechanical Systems in the Quantum Regime
Introduction
Quantum computing is a field of study focused on the development of computer technologies centered around the principles of quantum theory. Quantum theory explains the nature and behavior of energy and matter on the quantum (atomic and subatomic) level. Quantum computing involves developing computer technology based on the principles of quantum theory, which explains the behavior of energy and matter on the quantum (atomic and subatomic) level. Quantum computing involves developing computer technology based on the principles of quantum theory.
Quantum optomechanical systems are devices that allow for the study and manipulation of mechanical vibrations at the quantum level. These systems are typically composed of a mechanical oscillator, such as a tiny mirror or a vibrating drum, coupled to a light field. The interaction between the light and the mechanical oscillator can lead to a variety of interesting quantum phenomena, such as quantum entanglement and quantum superposition.
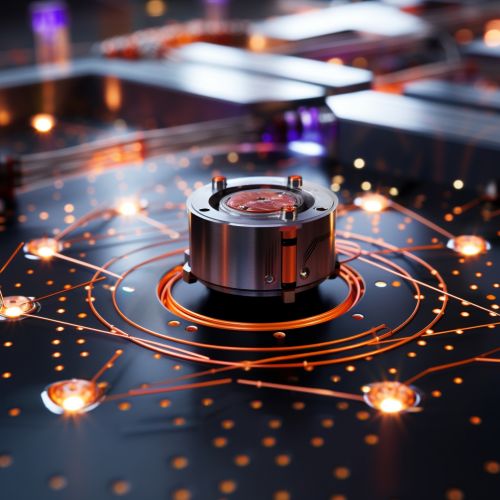
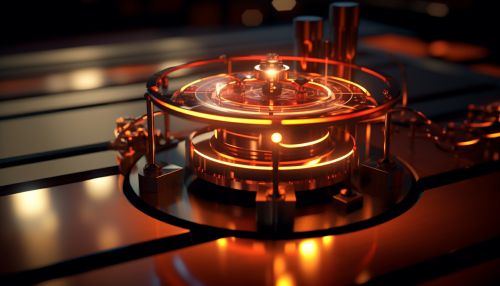
Quantum Optomechanical Systems
Quantum optomechanical systems are a type of quantum system that involves the interaction of light with mechanical motion. These systems can be used to study a variety of quantum phenomena, such as quantum entanglement and quantum superposition, and they have potential applications in areas such as quantum computing and quantum information processing.
The basic components of a quantum optomechanical system are a mechanical oscillator and a light field. The mechanical oscillator can be a tiny mirror or a vibrating drum, and the light field is typically provided by a laser. The interaction between the light and the mechanical oscillator can lead to a variety of interesting quantum phenomena.
One of the key features of quantum optomechanical systems is the ability to cool the mechanical oscillator to its quantum ground state. This is achieved by using the light field to exert a force on the mechanical oscillator, which can be used to dampen its motion. This process, known as optomechanical cooling, is crucial for the study of quantum phenomena in these systems.
Quantum optomechanical systems also allow for the manipulation of mechanical vibrations at the quantum level. This is achieved by using the light field to exert a force on the mechanical oscillator, which can be used to drive its motion. This process, known as optomechanical driving, is crucial for the manipulation of quantum states in these systems.
Quantum Computing with Quantum Optomechanical Systems
Quantum computing with quantum optomechanical systems involves using these systems to perform quantum computations. This can be achieved by using the mechanical oscillator as a quantum bit, or qubit, which is the basic unit of quantum information.
In a quantum computer, the qubits are used to store and manipulate information in a way that is fundamentally different from classical computers. Instead of bits, which can be either 0 or 1, qubits can be in a superposition of states, meaning they can be both 0 and 1 at the same time. This allows quantum computers to perform complex calculations much more efficiently than classical computers.
Quantum optomechanical systems provide a unique platform for quantum computing, as they allow for the manipulation of mechanical vibrations at the quantum level. This can be used to perform quantum operations on the qubits, such as quantum gates, which are the basic building blocks of a quantum computer.
One of the key challenges in quantum computing with quantum optomechanical systems is the need to cool the mechanical oscillator to its quantum ground state. This is necessary in order to minimize the effects of thermal noise, which can lead to errors in the quantum computations. However, this can be achieved using optomechanical cooling, which is a well-established technique in the field of quantum optomechanics.
Quantum Regime
The quantum regime refers to the range of parameters for which quantum effects become significant. In the context of quantum optomechanical systems, this typically means that the mechanical oscillator is cooled to its quantum ground state and that the interaction between the light and the mechanical oscillator is strong enough to induce quantum effects.
In the quantum regime, quantum optomechanical systems can exhibit a variety of interesting phenomena, such as quantum entanglement and quantum superposition. These phenomena can be used to perform quantum computations, making quantum optomechanical systems a promising platform for quantum computing.
One of the key challenges in reaching the quantum regime is the need to cool the mechanical oscillator to its quantum ground state. This is necessary in order to minimize the effects of thermal noise, which can lead to errors in the quantum computations. However, this can be achieved using optomechanical cooling, which is a well-established technique in the field of quantum optomechanics.
Conclusion
Quantum computing with quantum optomechanical systems in the quantum regime is a promising field of study. These systems provide a unique platform for quantum computing, as they allow for the manipulation of mechanical vibrations at the quantum level. This can be used to perform quantum operations on the qubits, such as quantum gates, which are the basic building blocks of a quantum computer.
One of the key challenges in this field is the need to cool the mechanical oscillator to its quantum ground state. This is necessary in order to minimize the effects of thermal noise, which can lead to errors in the quantum computations. However, this can be achieved using optomechanical cooling, which is a well-established technique in the field of quantum optomechanics.
Despite these challenges, the potential benefits of quantum computing with quantum optomechanical systems are significant. Quantum computers have the potential to solve problems that are intractable for classical computers, and quantum optomechanical systems provide a unique platform for realizing this potential.