Quantum Computing with Quantum Optomechanical Systems in Various Quantum Regimes
Introduction
Quantum computing is a rapidly developing field that leverages the principles of quantum mechanics to process information. One of the promising platforms for implementing quantum computing is Quantum Optomechanical Systems (QOMS). These systems couple mechanical oscillators to optical fields, enabling the manipulation of quantum states. This article delves into the use of QOMS in various quantum regimes for quantum computing.
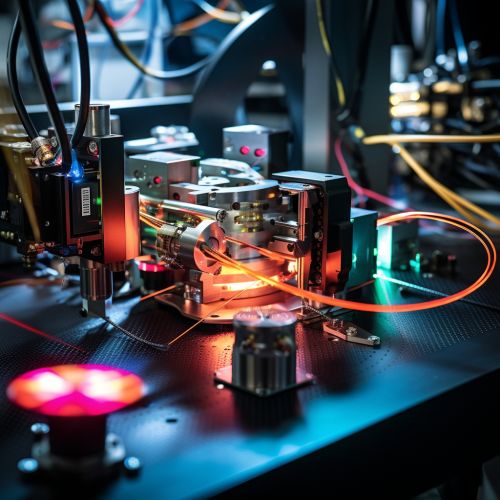
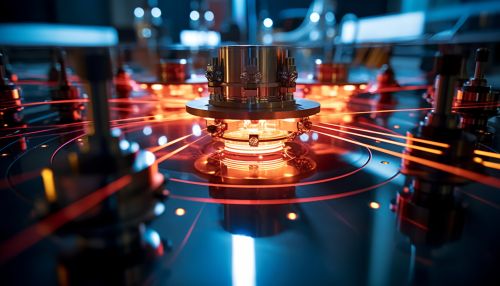
Quantum Optomechanical Systems
Quantum Optomechanical Systems (QOMS) are physical systems where the mechanical motion of an object is coupled to the electromagnetic field of a cavity. The interaction between light and mechanical motion in these systems can be harnessed to control and manipulate quantum states, making them a promising platform for quantum computing.
Principles of Operation
The operation of QOMS is based on the radiation pressure exerted by light. When light is confined in a cavity, it exerts a force on the mirrors of the cavity. This force can cause the mirrors to move, leading to a change in the cavity length. The change in cavity length, in turn, affects the resonance condition of the cavity, leading to a change in the light field. This interaction between light and mechanical motion forms the basis of optomechanical coupling.
Types of Quantum Optomechanical Systems
There are several types of QOMS, each with its unique characteristics and applications. Some of the most common types include:
- Optomechanical Crystals: These are structures that confine both light and mechanical vibrations to the same region, enabling strong optomechanical coupling.
- Photonic Crystal Cavities: These are cavities formed by periodic variations in the refractive index of a material, enabling the confinement of light and mechanical motion.
- Nano-Mechanical Resonators: These are tiny mechanical structures that can oscillate at very high frequencies, enabling the manipulation of quantum states.
Quantum Regimes
In the context of QOMS, quantum regimes refer to the different conditions under which the systems can operate. These regimes are defined by the strength of the optomechanical coupling and the thermal occupation of the mechanical mode.
Ground State Cooling
Ground state cooling refers to the regime where the mechanical oscillator is cooled to its quantum ground state. This is achieved by using a strong laser drive to cool the mechanical mode to a temperature where its average occupation number is less than one.
Strong Coupling Regime
The strong coupling regime is characterized by a strong interaction between the light and mechanical motion, such that the optomechanical coupling rate exceeds the decay rates of the cavity and mechanical mode. In this regime, the quantum nature of the mechanical oscillator becomes apparent, and quantum effects such as quantum entanglement and quantum superposition can be observed.
Single-Photon Strong Coupling Regime
In the single-photon strong coupling regime, the optomechanical coupling rate exceeds the decay rates even at the single-photon level. This regime is particularly interesting for quantum computing, as it allows for the manipulation of quantum states at the single-photon level.
Quantum Computing with Quantum Optomechanical Systems
Quantum computing with QOMS involves using the optomechanical interaction to manipulate quantum states and perform quantum operations. This can be achieved in various quantum regimes, each offering unique advantages and challenges.
Quantum Gates
Quantum gates are fundamental operations in quantum computing. In QOMS, quantum gates can be implemented by using the optomechanical interaction to manipulate the quantum states of the mechanical oscillator. For example, a quantum NOT gate can be implemented by flipping the quantum state of the oscillator.
Quantum Error Correction
Quantum error correction is a crucial aspect of quantum computing, as it allows for the correction of errors that occur during quantum operations. In QOMS, quantum error correction can be achieved by using the optomechanical interaction to monitor the quantum state of the mechanical oscillator and correct any deviations from the desired state.
Quantum Communication
QOMS can also be used for quantum communication, which involves the transmission of quantum information over long distances. This can be achieved by using the optomechanical interaction to convert quantum states from the optical domain to the mechanical domain and vice versa, enabling the transmission of quantum information via mechanical vibrations.