Quantum Computing with Quantum Optomechanical Resonators
Introduction
Quantum computing is a rapidly evolving field that leverages the principles of quantum mechanics to perform computations. One of the key components of quantum computing systems is the quantum optomechanical resonator, a device that couples optical and mechanical oscillations at the quantum level. This article delves into the principles, applications, and challenges of quantum computing with quantum optomechanical resonators.
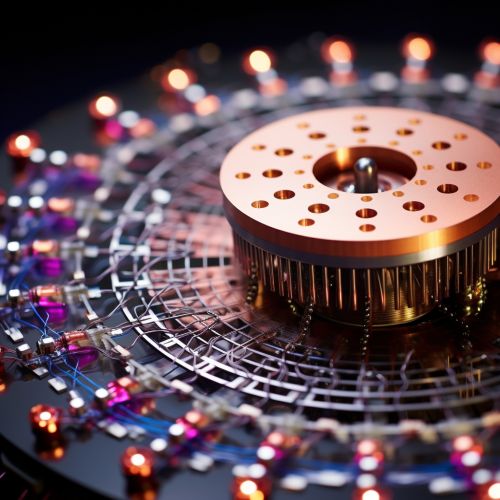
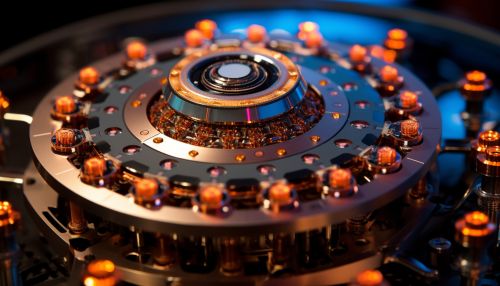
Quantum Optomechanical Resonators
Quantum optomechanical resonators are devices that couple light (optical oscillations) and mechanical motion at the quantum level. This coupling is achieved through radiation pressure, the force exerted by light on a surface. In these resonators, the radiation pressure of light can cause mechanical oscillations, which in turn modulate the light's properties. This interplay between light and motion is the essence of optomechanical coupling.
Principles
The principles of quantum optomechanical resonators are rooted in the laws of quantum mechanics. The key concept is the quantum superposition principle, which allows quantum systems to exist in multiple states simultaneously. In the context of optomechanical resonators, this principle enables the simultaneous oscillation of the resonator in multiple mechanical states.
Another important principle is the quantum entanglement, which allows particles to be interconnected such that the state of one particle instantly influences the state of the other, regardless of the distance between them. In quantum optomechanical resonators, entanglement can be achieved between the mechanical and optical modes, leading to a variety of quantum phenomena.
Design and Fabrication
The design and fabrication of quantum optomechanical resonators involve several steps, including material selection, resonator design, and fabrication process. The material must have a high mechanical quality factor and low optical loss. The resonator design must ensure strong optomechanical coupling, which is typically achieved by maximizing the overlap between the optical and mechanical modes. The fabrication process involves techniques such as lithography and etching, which must be carefully controlled to ensure the resonator's performance.
Quantum Computing with Quantum Optomechanical Resonators
Quantum optomechanical resonators have found significant applications in quantum computing. They serve as quantum bits (qubits), the fundamental units of information in quantum computing. Unlike classical bits, which can be either 0 or 1, qubits can exist in a superposition of states, enabling quantum computers to process a vast amount of information simultaneously.
Quantum Information Processing
In quantum information processing, quantum optomechanical resonators can be used to store, manipulate, and transfer quantum information. The optomechanical interaction allows the conversion of quantum information between different forms, such as from optical to mechanical and vice versa. This capability is crucial for quantum communication, where quantum information needs to be transferred over long distances.
Quantum Error Correction
Quantum error correction is a critical aspect of quantum computing, as quantum systems are highly susceptible to errors due to environmental disturbances. Quantum optomechanical resonators can be used to implement quantum error correction codes, which protect the quantum information from errors without disturbing the quantum state.
Challenges and Future Directions
Despite the significant progress in quantum computing with quantum optomechanical resonators, several challenges remain. These include the efficient generation and detection of quantum states, the realization of strong optomechanical coupling, and the mitigation of decoherence, the process that causes quantum systems to lose their quantum properties.
Future research directions include the development of novel materials and designs for quantum optomechanical resonators, the exploration of new quantum phenomena, and the integration of quantum optomechanical resonators into large-scale quantum computing systems.