Quantum Computing with Quantum Information Processing
Introduction
Quantum computing is a field of study focused on the development and application of quantum computers, which are designed to use quantum bits, or "qubits", rather than the binary digits, or "bits", used in classical computing. Quantum computers leverage the principles of quantum mechanics to process information. Quantum Information Processing (QIP) is a subfield of quantum computing that studies how quantum systems can store, manipulate, and transmit information.
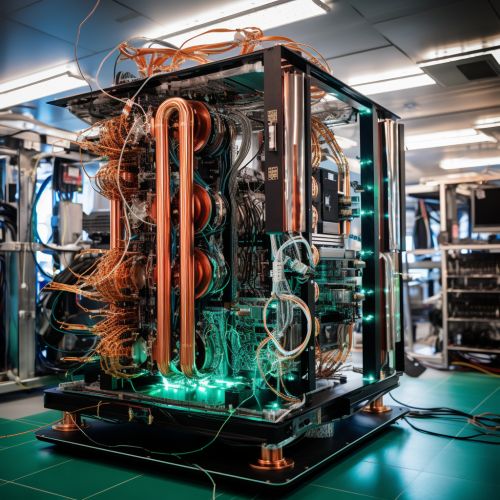
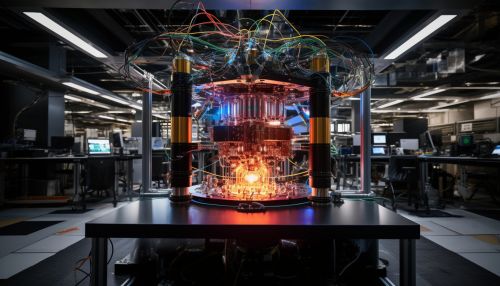
Quantum Mechanics and Quantum Computing
Quantum mechanics is the branch of physics that deals with the smallest particles in the universe, such as atoms and subatomic particles like electrons and photons. Quantum mechanics is fundamentally different from classical physics, and it introduces several new concepts that are essential to quantum computing.
One of the key principles of quantum mechanics is superposition, which allows quantum systems to exist in multiple states simultaneously. This is a stark contrast to classical systems, which can only exist in one state at a time. In the context of quantum computing, superposition allows a qubit to be in a state of 0, 1, or both at the same time.
Another important principle is entanglement, which is a phenomenon where two or more quantum particles become interconnected and the state of one particle can instantaneously affect the state of the other, regardless of the distance between them. This property is used in quantum computing to link qubits together, allowing for more complex computations.
Quantum Bits (Qubits)
In classical computing, information is processed using bits that can exist in one of two states: 0 or 1. Quantum computing, however, uses quantum bits, or qubits. A qubit can exist not only in a state corresponding to the logical state 0 or 1 as in a classical bit, but also in states corresponding to a blend or superposition of these classical states. This property allows quantum computers to process a vast number of computations simultaneously.
Qubits can be implemented using a variety of physical systems, such as atoms, ions, photons, and superconducting circuits. The choice of system can affect the computational properties of the quantum computer, including its speed, power, and reliability.
Quantum Gates and Circuits
Quantum gates are the basic building blocks of quantum circuits. They are operations that can be applied to a set of qubits, changing their quantum state. Quantum gates are analogous to the logic gates used in classical computing but have some crucial differences due to the principles of quantum mechanics.
Quantum circuits are sequences of quantum gates. The computation ends with a measurement, collapsing the system of qubits into one of the 2^n possible states, where n is the number of qubits used. The outcome of a measurement is used as the result of the computation.
Quantum Information Processing (QIP)
Quantum Information Processing (QIP) is a subfield of quantum computing that focuses on how quantum systems can be used to store, manipulate, and transmit information. It encompasses both quantum communication, which involves transmitting quantum information from one location to another, and quantum computation, which involves performing calculations using quantum systems.
QIP leverages the principles of quantum mechanics to perform tasks that are difficult or impossible for classical systems. For example, quantum algorithms, such as Shor's algorithm for factorizing large numbers and Grover's algorithm for searching unsorted databases, can solve certain problems more efficiently than their classical counterparts.
Quantum Error Correction
Quantum error correction is a set of techniques used to protect quantum information from errors due to decoherence and other quantum noise. Quantum error correction is essential for practical quantum computing, as quantum information is extremely sensitive to environmental noise.
Quantum error correction codes are used to detect and correct errors in quantum data. These codes work by encoding a logical qubit in a larger number of physical qubits. If an error occurs in one of the physical qubits, it can be detected and corrected without disturbing the information stored in the logical qubit.
Quantum Computing Applications
Quantum computing has the potential to revolutionize many areas of technology. Some of the most promising applications of quantum computing include cryptography, optimization, machine learning, and the simulation of quantum systems.
In cryptography, quantum computers could potentially break many of the encryption algorithms currently in use. However, they could also lead to the development of new, more secure cryptographic techniques, a field known as post-quantum cryptography.
In optimization and machine learning, quantum algorithms could potentially solve complex problems more efficiently than classical algorithms. This could have applications in a wide range of fields, including logistics, finance, and artificial intelligence.
In the simulation of quantum systems, quantum computers could provide insights into the behavior of quantum systems that are difficult to simulate classically. This could have applications in fields such as chemistry and materials science.
Conclusion
Quantum computing and Quantum Information Processing (QIP) represent a significant shift from classical computing. Leveraging the principles of quantum mechanics, these fields promise to revolutionize various areas of technology and science. While there are still many challenges to overcome, the potential benefits of quantum computing and QIP are immense.