Quantum Anomalous Hall Effect
Introduction
The Quantum Anomalous Hall Effect (QAHE) is a phenomenon in condensed matter physics that is a quantum version of the classical Anomalous Hall Effect. It was first predicted in the 1980s and observed experimentally in 2013. The QAHE is a manifestation of topological order, a new kind of quantum mechanical phase of matter. It is characterized by a quantized Hall conductance, which is independent of the details of the material and only depends on fundamental constants of nature.
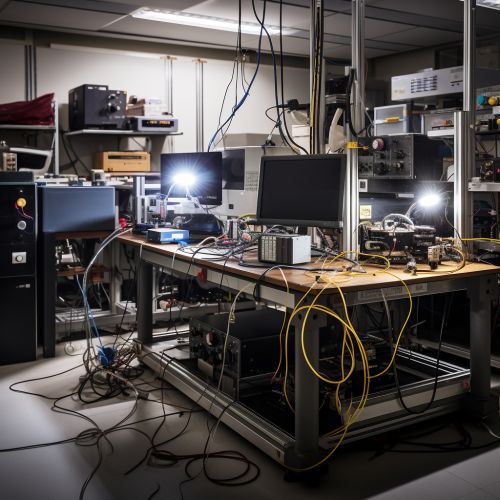
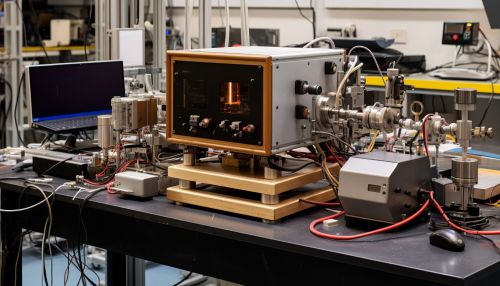
Theoretical Background
The QAHE is a consequence of the interplay between quantum mechanics and spin-orbit coupling, a relativistic effect that couples an electron's spin to its orbital motion. This coupling can lead to a band structure with non-trivial topology, which gives rise to the QAHE.
The theoretical foundation of the QAHE is rooted in the field of topological insulators, a class of materials that have an insulating interior but conductive surface states. These surface states are protected by time-reversal symmetry and cannot be removed by disorder or impurities. The QAHE can be understood as a result of breaking time-reversal symmetry in a topological insulator, which leads to a gap opening in the surface states and a quantized Hall conductance.
Experimental Observation
The first experimental observation of the QAHE was reported in thin films of chromium-doped (Bi,Sb)2Te3, a magnetic topological insulator. The researchers observed a quantized Hall resistance, a hallmark of the QAHE, at zero magnetic field. This was a significant breakthrough as it confirmed the theoretical predictions of the QAHE and demonstrated a new quantum state of matter.
Subsequent experiments have further confirmed the QAHE and explored its properties in various materials. These studies have revealed a rich variety of phenomena, including the coexistence of the QAHE with superconductivity and the observation of the QAHE at high temperatures.
Implications and Applications
The QAHE has profound implications for our understanding of quantum physics and could lead to new technologies. The quantized Hall conductance is a topological invariant, which means it is robust against perturbations. This makes devices based on the QAHE potentially useful for quantum computing, where the fragility of quantum states is a major challenge.
In addition, the QAHE could be used to create low-power electronic devices. Unlike conventional electronics, which rely on the flow of charge, devices based on the QAHE could operate using the spin of electrons, a concept known as spintronics. This could lead to more energy-efficient electronics.
Future Directions
While the QAHE has been observed experimentally, many questions remain. For example, the mechanism behind the high-temperature QAHE is not fully understood. In addition, the search for new materials that exhibit the QAHE is an active area of research. These challenges, and others, will continue to drive research in this exciting field.