Protein–RNA complex
Introduction
A protein–RNA complex is a molecular assembly consisting of one or more protein molecules bound to one or more RNA molecules. These complexes play crucial roles in various biological processes, including gene expression, RNA splicing, translation, and RNA interference. Understanding the structure and function of protein–RNA complexes is essential for elucidating the mechanisms of cellular regulation and for developing therapeutic strategies targeting these interactions.
Structure of Protein–RNA Complexes
Protein–RNA complexes exhibit a wide range of structural diversity, reflecting their varied functions. The structure of these complexes can be studied using techniques such as X-ray crystallography, nuclear magnetic resonance (NMR) spectroscopy, and cryogenic electron microscopy (cryo-EM). These methods provide high-resolution details of the interactions between proteins and RNA molecules.
RNA-Binding Domains
Proteins that interact with RNA typically contain specific RNA-binding domains (RBDs). Some of the most well-characterized RBDs include the RNA recognition motif (RRM), the K homology domain (KH domain), and the zinc finger domains. Each of these domains recognizes specific RNA sequences or structures, facilitating the formation of stable protein–RNA complexes.
RNA Structures
RNA molecules within protein–RNA complexes often adopt intricate secondary and tertiary structures. Common RNA structural motifs include stem-loops, hairpin loops, and pseudoknots. These structures are critical for the specific recognition by RNA-binding proteins and for the overall stability of the complex.
Functions of Protein–RNA Complexes
Protein–RNA complexes are involved in a myriad of cellular processes. Below are some of the key functions:
Gene Expression Regulation
Protein–RNA complexes play a pivotal role in the regulation of gene expression. For example, the spliceosome is a large protein–RNA complex responsible for RNA splicing, a process that removes introns from pre-mRNA transcripts. Another example is the RNA-induced silencing complex (RISC), which mediates RNA interference by binding to microRNAs (miRNAs) or small interfering RNAs (siRNAs) and guiding them to complementary mRNA targets for degradation.
Translation
In the context of translation, the ribosome is a quintessential protein–RNA complex. It consists of ribosomal RNA (rRNA) and ribosomal proteins, and it facilitates the synthesis of proteins by translating the genetic code carried by mRNA. The signal recognition particle (SRP) is another protein–RNA complex that directs nascent polypeptides to the endoplasmic reticulum for proper folding and processing.
RNA Modification
Protein–RNA complexes are also involved in the post-transcriptional modification of RNA. The small nucleolar ribonucleoprotein (snoRNP) complexes, for instance, guide the chemical modification of rRNA, such as 2'-O-methylation and pseudouridylation. These modifications are essential for the proper function of the ribosome.
Mechanisms of Protein–RNA Interaction
The interaction between proteins and RNA is governed by several mechanisms, including sequence-specific recognition, structural complementarity, and electrostatic interactions.
Sequence-Specific Recognition
Many RNA-binding proteins recognize specific RNA sequences through direct base-pairing interactions. For example, the Pumilio and FBF homology (PUF) proteins bind to specific RNA sequences via a series of repeat motifs that interact with individual RNA bases.
Structural Complementarity
In addition to sequence-specific interactions, structural complementarity between the protein and RNA is crucial for complex formation. The three-dimensional shapes of RNA-binding domains and RNA structural motifs must fit together precisely to form a stable complex.
Electrostatic Interactions
Electrostatic interactions also play a significant role in protein–RNA binding. RNA molecules are negatively charged due to their phosphate backbone, and many RNA-binding proteins contain positively charged regions that can interact with the RNA. These electrostatic interactions help stabilize the protein–RNA complex.
Techniques for Studying Protein–RNA Complexes
Several advanced techniques are employed to study the structure and function of protein–RNA complexes.
X-ray Crystallography
X-ray crystallography is a powerful technique for determining the atomic structure of protein–RNA complexes. By crystallizing the complex and analyzing the diffraction pattern of X-rays passing through the crystal, researchers can build detailed models of the complex's structure.
Nuclear Magnetic Resonance (NMR) Spectroscopy
Nuclear magnetic resonance (NMR) spectroscopy provides information about the structure and dynamics of protein–RNA complexes in solution. NMR can be used to study complexes that are difficult to crystallize, offering insights into their conformational flexibility and interaction dynamics.
Cryogenic Electron Microscopy (Cryo-EM)
Cryogenic electron microscopy (cryo-EM) has emerged as a revolutionary technique for studying large protein–RNA complexes at near-atomic resolution. Cryo-EM involves rapidly freezing the complex and imaging it with an electron microscope, allowing researchers to visualize its structure in a native-like state.
Biological Implications and Applications
Understanding protein–RNA complexes has significant implications for biology and medicine.
Disease Mechanisms
Dysregulation of protein–RNA interactions is implicated in various diseases. For example, mutations in RNA-binding proteins can lead to neurodegenerative diseases such as amyotrophic lateral sclerosis (ALS) and frontotemporal dementia (FTD). Aberrant protein–RNA complexes are also involved in cancer and viral infections.
Therapeutic Targeting
Protein–RNA complexes are attractive targets for therapeutic intervention. Small molecules, antisense oligonucleotides, and RNA interference (RNAi) strategies can be designed to disrupt or modulate specific protein–RNA interactions. For instance, Spinraza (nusinersen) is an antisense oligonucleotide used to treat spinal muscular atrophy by modifying the splicing of the SMN2 gene.
Future Directions
Research on protein–RNA complexes is rapidly advancing, with several promising directions for future studies.
High-Throughput Techniques
The development of high-throughput techniques, such as CLIP-seq (crosslinking and immunoprecipitation sequencing) and RIP-seq (RNA immunoprecipitation sequencing), allows for the genome-wide identification of RNA targets of RNA-binding proteins. These techniques provide a comprehensive view of the protein–RNA interactome.
Structural Dynamics
Understanding the dynamic nature of protein–RNA complexes is crucial for elucidating their function. Advanced techniques such as single-molecule fluorescence resonance energy transfer (smFRET) and time-resolved cryo-EM are being used to study the conformational changes and interaction kinetics of these complexes.
Computational Modeling
Computational approaches, including molecular dynamics simulations and machine learning algorithms, are increasingly being used to predict and analyze protein–RNA interactions. These methods complement experimental techniques and provide valuable insights into the mechanisms of complex formation.
See Also
- Gene expression
- RNA splicing
- Translation (biology)
- RNA interference
- Ribosome
- Signal recognition particle
- Small nucleolar ribonucleoprotein
- Nuclear magnetic resonance spectroscopy
- Cryogenic electron microscopy
- Neurodegenerative diseases
- Cancer
- Viral infections
- RNA interference
- Spinraza
- CLIP-seq
- RIP-seq
- Single-molecule fluorescence resonance energy transfer
- Molecular dynamics simulations
- Machine learning
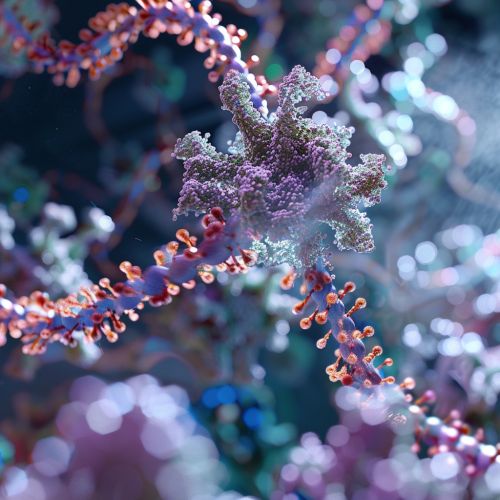
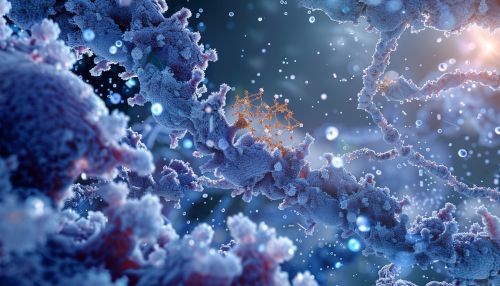