Positron emission tomography
Introduction
Positron emission tomography (PET) is a nuclear medicine functional imaging technique that produces a three-dimensional image of functional processes in the body. The system detects pairs of gamma rays emitted indirectly by a positron-emitting radionuclide, also known as a tracer, which is introduced into the body on a biologically active molecule. PET is both a medical and research tool, and is used heavily in clinical oncology and for clinical diagnosis of certain diffuse brain diseases.
History
The concept of PET was first proposed by James S. Robertson and his student William G. Hoffman in the late 1950s. The first PET scanner was built in 1961 by Robertson and Hoffman at the University of Pennsylvania. This early version of PET technology was crude and had limited capabilities, but it paved the way for the development of modern, sophisticated PET scanners.
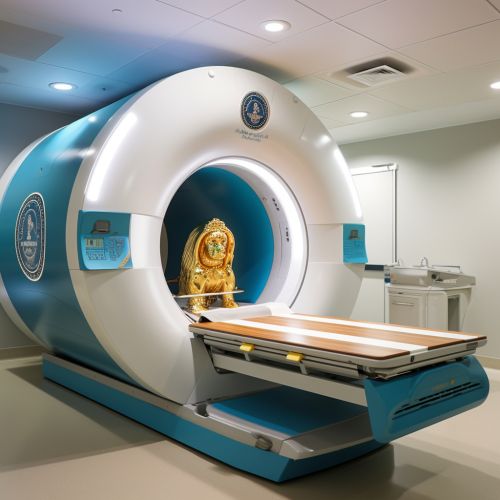
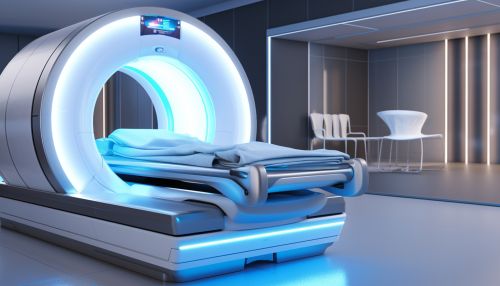
Principle of Operation
PET works on the principle of radioactive decay. The tracer used in PET is a biologically active molecule that has been labelled with a positron-emitting radionuclide. When this molecule undergoes radioactive decay, it emits a positron, which is an anti-particle of the electron. This positron travels a short distance before it encounters an electron, at which point the two particles annihilate each other and produce two gamma rays. These gamma rays are detected by the PET scanner, which uses this information to construct a three-dimensional image of the tracer concentration within the body.
Tracers
The most commonly used tracer in PET is fluorodeoxyglucose (FDG), a glucose analog. FDG is taken up by cells in proportion to their metabolic activity, making it a useful tracer for imaging tumors, which have a high metabolic rate. Other tracers are used for different purposes, such as 11C-labelled Pittsburgh Compound-B (PiB) for imaging beta-amyloid plaques in Alzheimer's disease.
Image Reconstruction
The raw data collected by a PET scanner consists of a list of 'coincidence events' representing near-simultaneous detection of two gamma rays by the scanner. This data is then processed to produce a sinogram, which is a representation of the tracer concentration as a function of position and angle. The sinogram is then reconstructed into an image using one of several available algorithms.
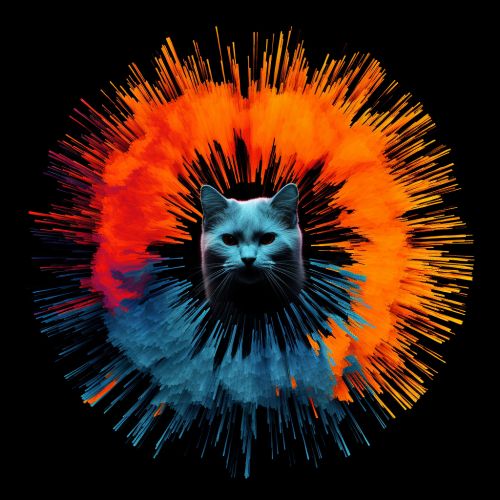
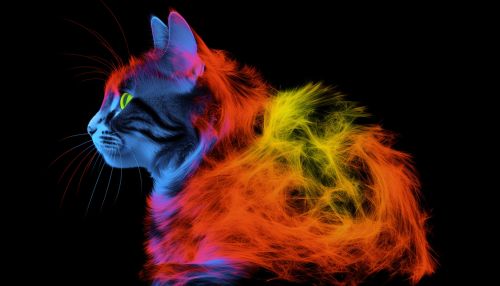
Clinical Applications
PET has a wide range of clinical applications, including oncology, cardiology, and neurology. In oncology, PET is used to detect and stage tumors, guide biopsy and resection, and monitor response to therapy. In cardiology, PET can be used to assess myocardial perfusion, viability, and function. In neurology, PET is used to diagnose and stage neurodegenerative diseases, epilepsy, and brain tumors.
Research Applications
In addition to its clinical applications, PET is also a powerful research tool. It is used in studies of drug metabolism, neurochemistry, and functional brain imaging. PET can also be used in conjunction with other imaging modalities, such as magnetic resonance imaging (MRI), to provide more comprehensive information about the structure and function of the body.
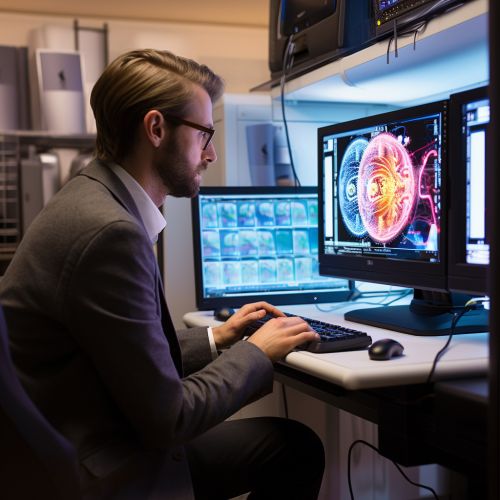
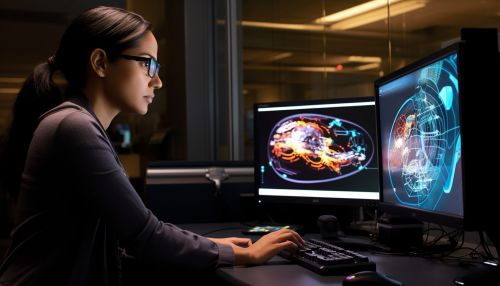
Limitations and Risks
While PET is a powerful imaging modality, it does have some limitations and risks. The resolution of PET is relatively low compared to other imaging modalities, and it is sensitive to patient movement. PET also exposes the patient to ionizing radiation, which carries a small risk of inducing cancer. However, the benefits of PET generally outweigh these risks in most clinical scenarios.
Future Directions
The field of PET is constantly evolving, with ongoing research into new tracers, improved image reconstruction algorithms, and novel applications. One promising area of research is the development of tracers for imaging specific molecular targets, which could greatly enhance the diagnostic and therapeutic capabilities of PET.