Planetary Boundary Layer in Atmospheric Science
Introduction
The Planetary Boundary Layer, also known as the Atmospheric Boundary Layer (ABL), is a part of the troposphere that is directly influenced by the presence of the Earth's surface, and responds to surface forcings with a timescale of about an hour or less. This layer in the atmosphere plays a crucial role in the transport of momentum, heat, and moisture, as well as the dispersion of pollutants.
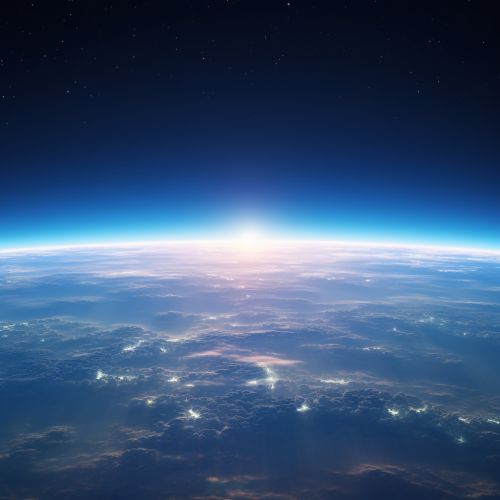
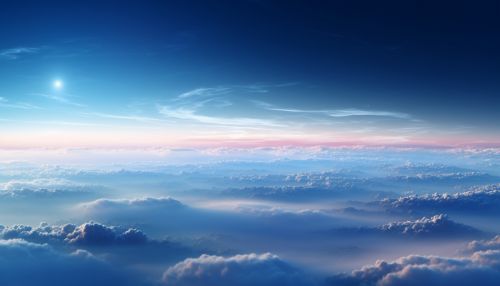
Structure of the Planetary Boundary Layer
The structure of the Planetary Boundary Layer varies significantly depending on the time of day, weather conditions, and the nature of the underlying surface. However, it can generally be divided into three sub-layers: the surface layer, the mixed layer, and the entrainment zone.
Surface Layer
The surface layer, also known as the constant flux layer, is the layer closest to the Earth's surface, typically extending up to about 10% of the total height of the PBL. In this layer, the mean flow is largely influenced by the friction with the Earth's surface, and the vertical gradients of wind speed, temperature, and moisture are typically large. The wind speed usually decreases towards the surface, a phenomenon known as the wind shear.
Mixed Layer
Above the surface layer is the mixed layer, also known as the convective layer or the Ekman layer. This layer extends from the top of the surface layer to the base of the entrainment zone. In this layer, the vertical gradients of wind speed, temperature, and moisture are typically small due to the strong vertical mixing, which is often driven by thermal convection.
Entrainment Zone
The entrainment zone, also known as the capping inversion or the inversion layer, is a thin layer located at the top of the PBL, where the temperature usually increases with height, contrary to the typical decrease in the troposphere. This layer acts as a barrier to the vertical movement of air, thus limiting the height of the PBL.
Dynamics of the Planetary Boundary Layer
The dynamics of the PBL are governed by a complex interplay of various physical processes, including turbulence, convection, radiation, and surface forcings. These processes result in a highly variable and turbulent flow within the PBL, which can have significant implications for the transport and dispersion of pollutants, the formation of clouds, and the development of weather systems.
Turbulence
Turbulence is a key feature of the PBL, and it plays a crucial role in the vertical mixing of momentum, heat, and moisture. Turbulence in the PBL is primarily generated by the wind shear at the surface and the thermal convection in the mixed layer. The turbulence intensity is typically highest in the surface layer and decreases with height.
Convection
Thermal convection is another important process in the PBL, especially during the daytime when the surface is heated by the sun. The heating of the surface creates buoyant air parcels, which rise and generate convective currents in the mixed layer. These convective currents enhance the vertical mixing and lead to the formation of thermals and convective clouds.
Radiation
The interaction between the PBL and the radiation from the sun and the Earth's surface also plays a significant role in the dynamics of the PBL. During the daytime, the absorption of solar radiation by the surface leads to the heating of the air in the surface layer, which drives the thermal convection. During the nighttime, the cooling of the surface by longwave radiation leads to the formation of a stable boundary layer, where the vertical mixing is suppressed.
Surface Forcings
The nature of the underlying surface can have a significant impact on the dynamics of the PBL. For example, the roughness of the surface can enhance the surface friction and thus the generation of turbulence. The type of surface (e.g., land, water, ice) can also affect the heat and moisture fluxes at the surface, which can influence the thermal convection and the stability of the PBL.
Measurement and Modeling of the Planetary Boundary Layer
The measurement and modeling of the PBL are essential for understanding its dynamics and its impact on the weather, climate, and air quality. Various observational techniques, such as weather balloons, radar, and lidar, are used to measure the properties of the PBL, including its height, temperature, humidity, wind speed, and turbulence intensity.
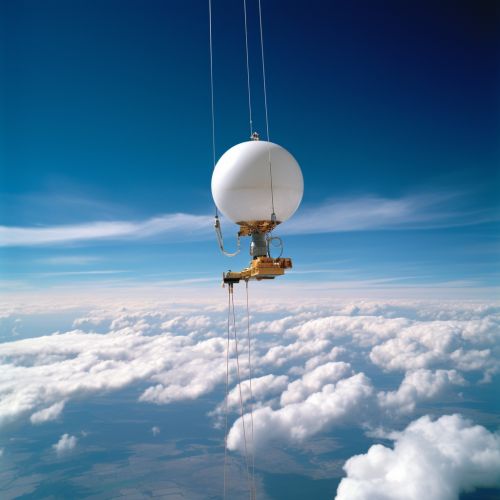
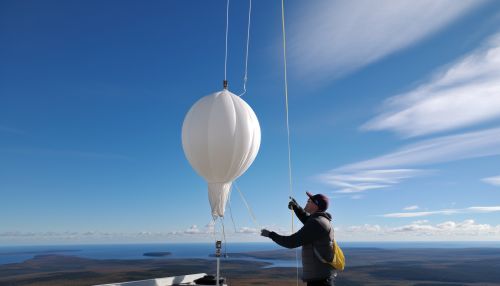
In addition to observations, numerical models are widely used to simulate the dynamics of the PBL. These models, which include both large-eddy simulations (LES) and weather prediction models, solve the fundamental equations of fluid dynamics and thermodynamics to predict the evolution of the PBL. However, due to the complexity of the PBL, these models often need to use simplifications and approximations, known as parameterizations, to represent the sub-grid scale processes, such as turbulence and convection.
Implications for Weather, Climate, and Air Quality
The PBL has significant implications for the weather, climate, and air quality. As the lowest part of the atmosphere, the PBL is where we live and breathe, and it is where most weather phenomena occur. The dynamics of the PBL can influence the formation of clouds, the development of weather systems, and the dispersion of pollutants.
Weather
The PBL plays a crucial role in the development of weather systems. For example, the thermal convection in the PBL can lead to the formation of convective clouds and thunderstorms. The turbulence in the PBL can also influence the wind speed and direction at the surface, which can affect the weather conditions.
Climate
The PBL also has a significant impact on the climate. The vertical mixing in the PBL can transport heat and moisture from the surface to the upper atmosphere, which can influence the global energy balance and the climate. The interaction between the PBL and the surface can also affect the land-atmosphere feedbacks, which can influence the climate sensitivity and the climate change.
Air Quality
The dynamics of the PBL can have a significant impact on the air quality. The turbulence in the PBL can enhance the dispersion of pollutants, thus reducing their concentrations. However, the stable boundary layer during the nighttime can trap the pollutants near the surface, leading to high pollution levels. The height of the PBL can also influence the vertical distribution of pollutants, which can affect their long-range transport and their impact on the air quality.