Physics of Superconductivity in Energy Transmission
Introduction
Superconductivity is a quantum mechanical phenomenon where certain materials exhibit zero electrical resistance and expulsion of magnetic fields when cooled below a characteristic critical temperature. This property makes them highly desirable for energy transmission applications, as it allows for the lossless transmission of electrical energy.
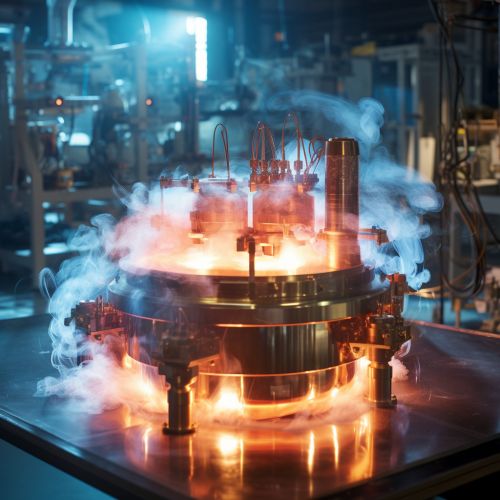
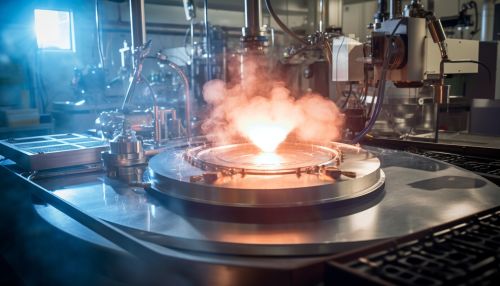
Discovery and History
The phenomenon of superconductivity was first discovered in 1911 by Heike Kamerlingh Onnes, who observed that the resistance of mercury dropped suddenly to zero at a temperature of approximately 4.2 Kelvin. This discovery led to a new field of study within physics, focusing on the properties and potential applications of superconductors.
Theoretical Understanding
The theoretical understanding of superconductivity has evolved over the years, with significant contributions from several key theories. The most notable among these is the BCS theory, named after its developers John Bardeen, Leon Cooper, and Robert Schrieffer. This theory explains superconductivity as a state in which electrons form pairs, known as Cooper pairs, that move through the lattice structure of the material without scattering off impurities and lattice vibrations, thus experiencing no resistance.
Types of Superconductors
Superconductors are typically classified into two types: Type I and Type II. Type I superconductors are pure metals and metalloids that exhibit superconductivity at very low temperatures. Type II superconductors, on the other hand, are usually metallic compounds or alloys that exhibit superconductivity at higher temperatures and can withstand higher magnetic fields before becoming normal conductors.
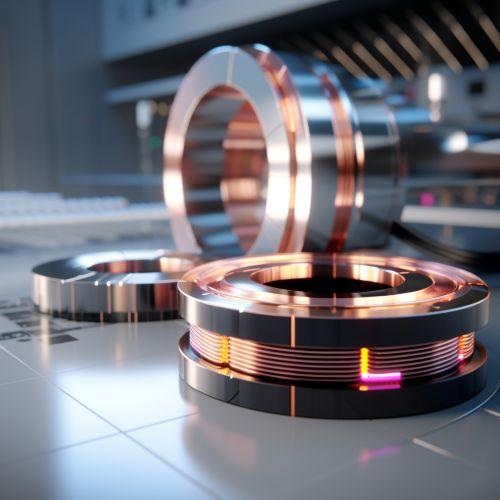
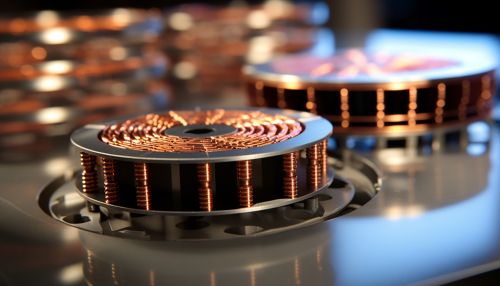
Superconductivity and Energy Transmission
The zero electrical resistance property of superconductors makes them ideal for energy transmission applications. Superconducting cables can transmit large amounts of electrical power without any loss, making them more efficient than conventional power cables. However, the practical implementation of superconducting cables in power grids is currently limited by several challenges, including the need for cooling to low temperatures and the high cost of superconducting materials.
High-Temperature Superconductors
A significant breakthrough in the field of superconductivity came with the discovery of high-temperature superconductors (HTS). These materials exhibit superconductivity at temperatures much higher than those required by traditional superconductors, making them more practical for real-world applications. However, the mechanism behind high-temperature superconductivity is still not fully understood, and it remains an active area of research in condensed matter physics.
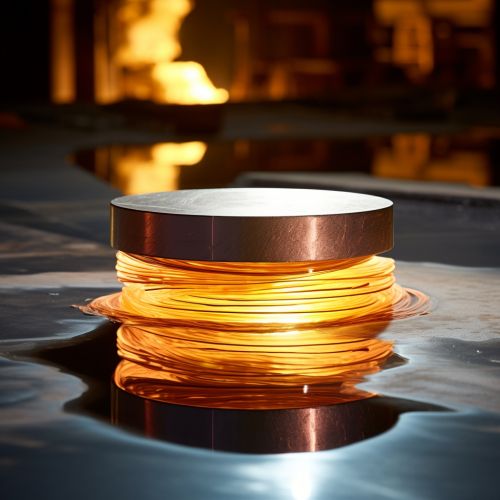
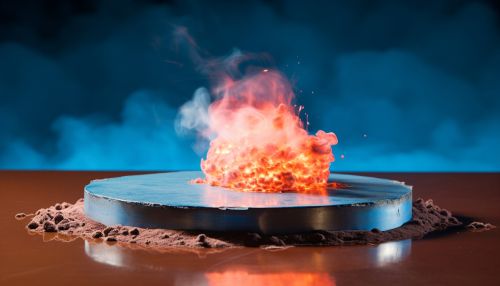
Future Prospects
The future of superconductivity in energy transmission looks promising, with ongoing research aimed at developing room-temperature superconductors and overcoming the challenges associated with the practical implementation of superconducting cables in power grids. If these challenges can be overcome, superconductors could revolutionize the field of energy transmission, leading to more efficient and sustainable power grids.