Oxidoreductase
Introduction
Oxidoreductases are a class of enzymes that catalyze oxidation-reduction reactions, where the transfer of electrons occurs between molecules. These enzymes play a crucial role in various biological processes, including cellular respiration, photosynthesis, and detoxification. Oxidoreductases are essential for maintaining the redox balance within cells and are involved in metabolic pathways that generate energy and synthesize biomolecules.
Classification
Oxidoreductases are classified based on the type of reaction they catalyze and the substrates involved. The Enzyme Commission (EC) number system categorizes oxidoreductases under EC 1, with further subdivisions based on specific reactions:
1. **EC 1.1**: Acting on the CH-OH group of donors. 2. **EC 1.2**: Acting on the aldehyde or oxo group of donors. 3. **EC 1.3**: Acting on the CH-CH group of donors. 4. **EC 1.4**: Acting on the CH-NH2 group of donors. 5. **EC 1.5**: Acting on the CH-NH group of donors. 6. **EC 1.6**: Acting on NADH or NADPH. 7. **EC 1.7**: Acting on other nitrogenous compounds as donors. 8. **EC 1.8**: Acting on a sulfur group of donors. 9. **EC 1.9**: Acting on a heme group of donors. 10. **EC 1.10**: Acting on diphenols and related substances as donors. 11. **EC 1.11**: Acting on peroxide as acceptor. 12. **EC 1.12**: Acting on hydrogen as donor. 13. **EC 1.13**: Acting on single donors with incorporation of molecular oxygen. 14. **EC 1.14**: Acting on paired donors with incorporation of molecular oxygen. 15. **EC 1.15**: Acting on superoxide radicals as acceptor. 16. **EC 1.16**: Oxidizing metal ions. 17. **EC 1.17**: Acting on CH or CH2 groups. 18. **EC 1.18**: Acting on iron-sulfur proteins as donors. 19. **EC 1.19**: Acting on reduced flavodoxin as donor. 20. **EC 1.20**: Acting on phosphorus or arsenic in donors. 21. **EC 1.21**: Acting on X-H and Y-H to form an X-Y bond. 22. **EC 1.97**: Other oxidoreductases.
Mechanism of Action
Oxidoreductases facilitate redox reactions by transferring electrons from a donor molecule (reductant) to an acceptor molecule (oxidant). The mechanism typically involves the formation of an enzyme-substrate complex, where the enzyme temporarily binds to the substrate. The enzyme then facilitates the transfer of electrons, resulting in the oxidation of the donor and the reduction of the acceptor. This process often involves cofactors such as NAD+, NADP+, FAD, or FMN, which act as electron carriers.
Structural Characteristics
The structure of oxidoreductases varies widely, reflecting their diverse functions. However, common features include active sites that accommodate specific substrates and cofactors. The active site often contains amino acid residues that participate in the redox reaction, such as cysteine, histidine, or tyrosine. Additionally, many oxidoreductases possess prosthetic groups, such as heme or iron-sulfur clusters, which facilitate electron transfer.
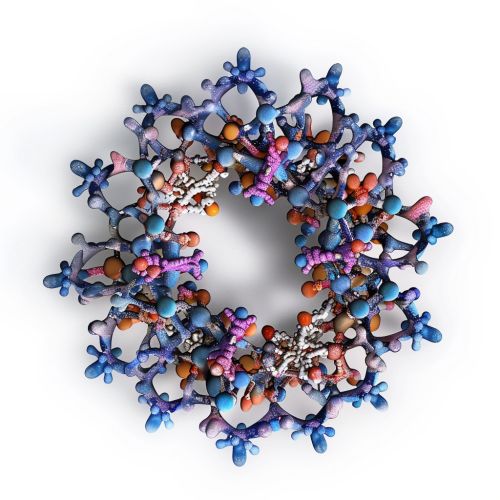
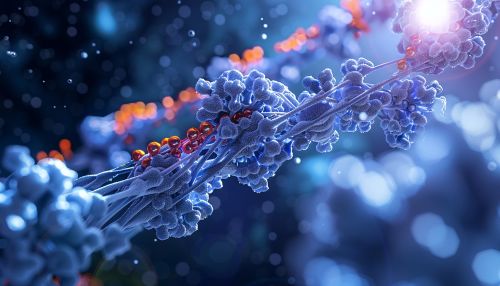
Biological Functions
Oxidoreductases are involved in numerous biological processes, including:
Cellular Respiration
In cellular respiration, oxidoreductases such as Cytochrome c oxidase and NADH dehydrogenase play critical roles in the electron transport chain, facilitating the transfer of electrons from NADH and FADH2 to molecular oxygen, ultimately generating ATP.
Photosynthesis
In photosynthesis, oxidoreductases like Photosystem II and Ferredoxin-NADP+ reductase are essential for the light-dependent reactions, where they facilitate the transfer of electrons from water to NADP+, producing NADPH and oxygen.
Detoxification
Oxidoreductases such as Cytochrome P450 enzymes are involved in the detoxification of xenobiotics and endogenous compounds. These enzymes catalyze the oxidation of substrates, making them more water-soluble and easier to excrete.
Antioxidant Defense
Enzymes like Superoxide dismutase and Catalase protect cells from oxidative damage by converting reactive oxygen species (ROS) into less harmful molecules. Superoxide dismutase catalyzes the dismutation of superoxide radicals into oxygen and hydrogen peroxide, while catalase converts hydrogen peroxide into water and oxygen.
Industrial Applications
Oxidoreductases have significant industrial applications due to their ability to catalyze specific redox reactions under mild conditions. Some notable applications include:
Biocatalysis
Oxidoreductases are used in biocatalysis to synthesize chiral compounds, which are important in the pharmaceutical industry. Enzymes like alcohol dehydrogenases and ketoreductases are employed to produce enantiomerically pure alcohols and ketones.
Biosensors
Oxidoreductases are integral components of biosensors used for detecting various analytes. For example, glucose oxidase is used in glucose sensors to monitor blood sugar levels in diabetic patients. The enzyme catalyzes the oxidation of glucose to gluconolactone, producing hydrogen peroxide, which is then detected electrochemically.
Environmental Biotechnology
Oxidoreductases are utilized in environmental biotechnology for the degradation of pollutants. Enzymes like laccases and peroxidases are employed in bioremediation processes to break down complex organic pollutants into less toxic compounds.
Research and Development
Ongoing research in the field of oxidoreductases focuses on understanding their structure-function relationships, engineering enzymes with enhanced properties, and discovering new oxidoreductases with unique catalytic activities. Advances in techniques such as X-ray crystallography and Cryo-electron microscopy have provided detailed insights into the three-dimensional structures of these enzymes, facilitating rational design and engineering efforts.
Challenges and Future Directions
Despite their potential, the industrial application of oxidoreductases faces several challenges, including enzyme stability, substrate specificity, and cost-effective production. Future research aims to address these challenges through protein engineering, directed evolution, and the development of robust expression systems. Additionally, the discovery of novel oxidoreductases from extremophiles and metagenomic libraries holds promise for expanding the repertoire of available enzymes.
See Also
- Enzyme
- Redox reaction
- Metabolism
- Electron transport chain
- Photosynthesis
- Biocatalysis
- Biosensor
- Bioremediation