Optical Lattices
Introduction
Optical lattices are periodic structures formed by the interference of counter-propagating laser beams, creating a spatially periodic polarization pattern. The resulting periodic potential may trap neutral atoms via the AC Stark shift, a phenomenon in which the frequency of an atom's electron transitions is shifted by the presence of an external electric field. This trapping of neutral atoms is a key aspect of the field of quantum simulation, and has potential applications in quantum computing and quantum information processing.
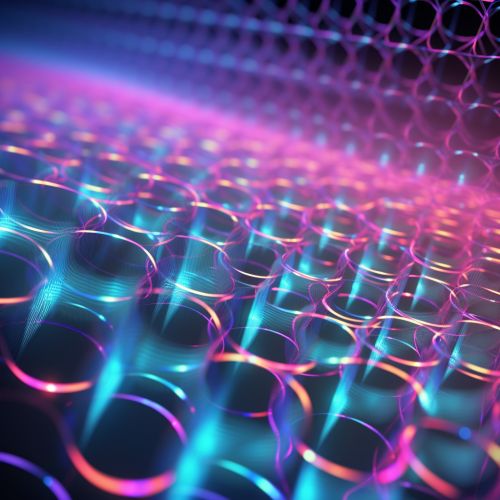
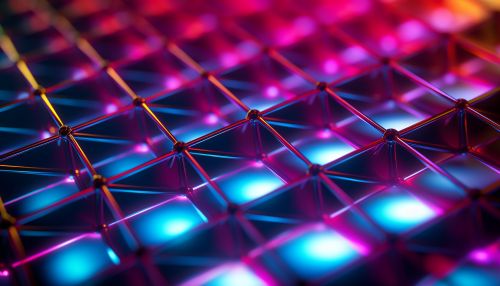
Formation of Optical Lattices
The formation of an optical lattice begins with the interference of two or more laser beams. When these beams are counter-propagating, they form a standing wave of light, which creates a spatially periodic polarization pattern. This pattern can be manipulated to form a lattice structure, with the nodes of the standing wave acting as potential wells that can trap neutral atoms.
The depth of these potential wells, and thus the strength of the optical lattice, can be controlled by adjusting the intensity of the laser beams. This allows for precise control over the properties of the optical lattice, including its periodicity and the number of atoms it can trap.
AC Stark Effect
The trapping of neutral atoms in an optical lattice is primarily due to the AC Stark effect. This effect, also known as the dynamic Stark effect, describes the shift in the frequency of an atom's electron transitions caused by an external electric field. In the context of optical lattices, the oscillating electric field of the laser light induces a time-dependent polarization in the neutral atoms, which leads to a shift in their energy levels.
The AC Stark effect can be either positive or negative, depending on the frequency of the laser light relative to the atomic transition frequency. If the laser frequency is higher (blue detuned), the atoms are attracted to the nodes of the standing wave, where the light intensity is lowest. If the laser frequency is lower (red detuned), the atoms are repelled from the nodes and attracted to the antinodes, where the light intensity is highest.
Applications
Optical lattices have a wide range of applications in the field of quantum simulation. By trapping atoms in a periodic potential, researchers can simulate the behavior of electrons in a solid, providing insights into phenomena such as superconductivity and quantum phase transitions.
In addition, optical lattices can be used to implement quantum gates for quantum computing. By manipulating the interactions between atoms in an optical lattice, it is possible to perform quantum operations that are the building blocks of a quantum computer.
Optical lattices also have potential applications in quantum information processing. The ability to trap and manipulate individual atoms makes optical lattices an ideal platform for storing and processing quantum information.