Molecular Pathways of Thermal Adaptation in Extremophiles
Introduction
Extremophiles are organisms that thrive in extreme environments, where most other life forms would not survive. One such extreme condition is high temperature, and the organisms that can survive in such conditions are known as thermophiles. The ability of these organisms to survive and thrive in high-temperature environments is due to their unique molecular pathways of thermal adaptation. This article will delve into the details of these molecular pathways, providing a comprehensive and detailed understanding of the subject.
Thermophiles
Thermophiles are a type of extremophile that thrive in high-temperature environments. These environments can range from hot springs to deep-sea hydrothermal vents, where temperatures can exceed 100 degrees Celsius. The ability of thermophiles to survive in these conditions is due to their unique molecular adaptations, which allow them to maintain the integrity of their cellular structures and functions despite the extreme heat.
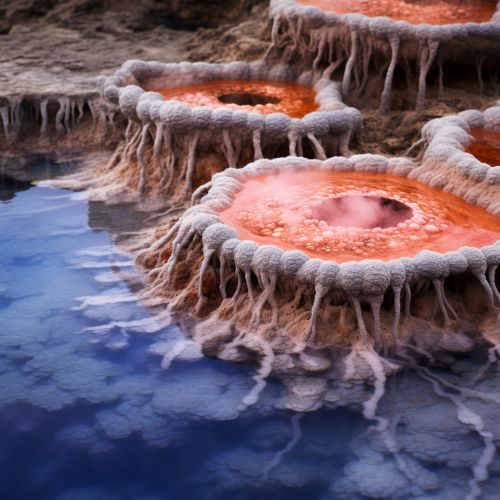
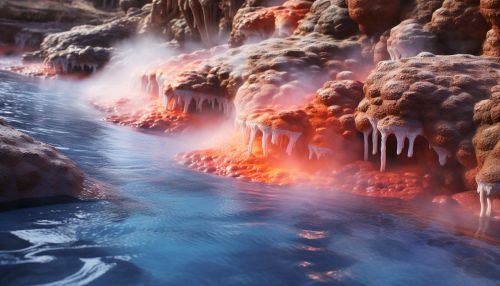
Molecular Adaptations
The molecular adaptations of thermophiles are diverse and complex, involving changes at the level of DNA, proteins, and cellular structures. Here, we will explore these adaptations in detail.
DNA Stability
One of the primary challenges faced by organisms in high-temperature environments is maintaining the stability of their DNA. High temperatures can cause DNA to denature, or separate into single strands, which can lead to mutations and cell death. Thermophiles have evolved several strategies to counteract this, including the use of DNA-binding proteins and modifications to the DNA itself.
DNA-Binding Proteins
Thermophiles produce a variety of DNA-binding proteins that help to stabilize the DNA molecule. These proteins bind to the DNA, preventing it from denaturing in the heat. Some of these proteins also have repair functions, helping to fix any damage that does occur.
DNA Modifications
In addition to DNA-binding proteins, thermophiles also modify their DNA to increase its stability. One common modification is the methylation of cytosine bases, which increases the melting temperature of the DNA and makes it more resistant to denaturation.
Protein Stability
Proteins are another major target of heat damage. High temperatures can cause proteins to denature, losing their three-dimensional structure and function. Thermophiles have evolved several strategies to counteract this, including the use of heat shock proteins and modifications to the proteins themselves.
Heat Shock Proteins
Heat shock proteins are a group of proteins that are produced in response to heat stress. These proteins help to refold denatured proteins, restoring their function. They also help to prevent protein aggregation, which can be lethal to the cell.
Protein Modifications
In addition to heat shock proteins, thermophiles also modify their proteins to increase their stability. These modifications can include changes in the amino acid sequence, the formation of disulfide bonds, and the addition of stabilizing molecules such as sugars.
Cellular Structures
Finally, thermophiles have adaptations at the level of their cellular structures. These include changes to the cell membrane and the cytoskeleton, which help to maintain the integrity of the cell in high-temperature environments.
Cell Membrane
The cell membrane of thermophiles is composed of lipids that are more saturated than those of mesophilic organisms. This increases the rigidity of the membrane, making it more resistant to heat damage.
Cytoskeleton
The cytoskeleton of thermophiles is also adapted to high temperatures. These organisms produce heat-stable versions of cytoskeletal proteins, which help to maintain the shape and integrity of the cell.
Conclusion
The molecular pathways of thermal adaptation in extremophiles are complex and diverse, involving changes at the level of DNA, proteins, and cellular structures. These adaptations allow thermophiles to survive and thrive in high-temperature environments, providing a fascinating example of the power of evolution. Further study of these pathways could provide insights into the limits of life on Earth, as well as potential applications in biotechnology and other fields.