Microbial Fuel Cells (MFCs)
Introduction
Microbial Fuel Cells (MFCs) are a type of bio-electrochemical system that harnesses the power of microorganisms to convert chemical energy, usually found in organic matter, into electrical energy. The concept of MFCs is not new, with initial experiments and theories dating back to the early 20th century. However, it is only in recent years that the technology has begun to gain significant attention due to its potential applications in various sectors, including wastewater treatment, energy production, and biosensors.
Principle of Operation
The basic principle of operation of MFCs lies in the metabolic processes of certain types of microorganisms. These microorganisms, often referred to as electrogenic bacteria, are capable of oxidizing organic matter and transferring the resulting electrons to an electrode. This process is often referred to as extracellular electron transfer (EET), and it forms the basis of electricity generation in MFCs.
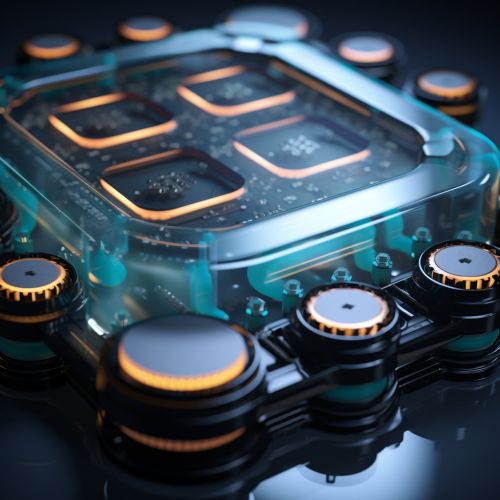
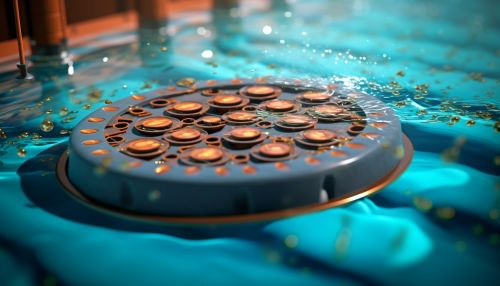
Components of MFCs
MFCs typically consist of two compartments, or cells, known as the anode and cathode, which are separated by a proton exchange membrane (PEM). Other key components include the microbial culture, which is usually situated in the anode compartment, and the electrodes.
Anode
The anode compartment of an MFC is where the oxidation of organic matter takes place. This process is facilitated by the electrogenic bacteria, which consume the organic matter as a source of energy and release electrons and protons as by-products. The electrons are transferred to the anode, while the protons pass through the PEM to the cathode compartment.
Cathode
In the cathode compartment, the electrons received from the anode, together with the protons that have passed through the PEM, combine with oxygen to form water. This process is known as reduction. The movement of electrons from the anode to the cathode via an external circuit is what generates electricity.
Proton Exchange Membrane
The PEM plays a crucial role in MFCs as it allows for the transfer of protons from the anode to the cathode, while preventing the direct mixing of the contents of the two compartments. This selective permeability of the PEM is essential for the efficient operation of MFCs.
Types of MFCs
There are several types of MFCs, classified based on factors such as the nature of the electrolyte, the type of microorganisms used, and the configuration of the cell. Some of the most common types include sediment MFCs, single-chamber MFCs, and dual-chamber MFCs.
Sediment MFCs
Sediment MFCs, also known as benthic MFCs, are typically used in environments where there is an abundance of organic matter, such as in sediments. The anode is buried in the sediment, while the cathode is placed in the overlying water, allowing for the natural potential difference between the two to be harnessed.
Single-Chamber MFCs
In single-chamber MFCs, the anode and cathode compartments are not physically separated. This design simplifies the construction of the MFC and eliminates the need for a PEM, but it also presents challenges in terms of maintaining the necessary conditions for the different reactions at the anode and cathode.
Dual-Chamber MFCs
Dual-chamber MFCs, as the name suggests, consist of two separate compartments for the anode and cathode, separated by a PEM. This design allows for greater control over the conditions in each compartment, but it also makes the MFC more complex and potentially more expensive to construct.
Applications of MFCs
The potential applications of MFCs are wide and varied, ranging from wastewater treatment to energy production and biosensors.
Wastewater Treatment
One of the most promising applications of MFCs is in the treatment of wastewater. The organic matter present in wastewater can be used as a source of energy for the electrogenic bacteria, while the electricity generated can be used to power the treatment process. This not only reduces the energy requirements of wastewater treatment, but also offers a potential solution for the disposal of organic waste.
Energy Production
MFCs can also be used as a source of renewable energy. The organic matter used by the bacteria can come from a variety of sources, including agricultural waste, food waste, and even human waste. The electricity generated can be used to power a range of devices, from small sensors to larger appliances.
Biosensors
The ability of MFCs to generate electricity from organic matter can also be used to create biosensors. By monitoring the electrical output of an MFC, it is possible to detect the presence of certain substances in the environment, such as pollutants or pathogens.
Challenges and Future Directions
Despite the potential benefits of MFCs, there are several challenges that need to be overcome before the technology can be widely adopted. These include improving the efficiency of electricity generation, scaling up the technology for industrial applications, and addressing issues related to the lifespan and durability of MFCs.
However, ongoing research in the field is promising, and there are several potential solutions to these challenges, including the development of new materials for electrodes and PEMs, the optimization of microbial cultures, and the design of more efficient cell configurations.