Gluconeogenesis
Introduction
Gluconeogenesis is a metabolic pathway that results in the generation of glucose from certain non-carbohydrate carbon substrates. This process is critical for maintaining blood glucose levels, especially during periods of fasting, starvation, or intense exercise. Gluconeogenesis primarily occurs in the liver and, to a lesser extent, in the renal cortex. This article delves deeply into the biochemical mechanisms, regulation, and physiological significance of gluconeogenesis.
Biochemical Mechanisms
Gluconeogenesis involves several key enzymes and intermediates that facilitate the conversion of substrates such as lactate, glycerol, and amino acids into glucose. The pathway is essentially the reverse of glycolysis, but with several key differences to ensure irreversibility and regulation.
Key Enzymes
- **Pyruvate Carboxylase**: This enzyme catalyzes the conversion of pyruvate to oxaloacetate in the mitochondria. It requires biotin as a cofactor and is activated by acetyl-CoA.
- **Phosphoenolpyruvate Carboxykinase (PEPCK)**: PEPCK converts oxaloacetate to phosphoenolpyruvate (PEP) in the cytoplasm. This reaction is driven by GTP.
- **Fructose-1,6-bisphosphatase**: This enzyme hydrolyzes fructose-1,6-bisphosphate to fructose-6-phosphate, bypassing the irreversible phosphofructokinase step in glycolysis.
- **Glucose-6-phosphatase**: This enzyme dephosphorylates glucose-6-phosphate to free glucose, which can then be released into the bloodstream.
Substrates
- **Lactate**: Produced by anaerobic glycolysis in muscles, lactate is transported to the liver where it is converted back to pyruvate by lactate dehydrogenase.
- **Glycerol**: Derived from the breakdown of triglycerides in adipose tissue, glycerol is phosphorylated to glycerol-3-phosphate and then oxidized to dihydroxyacetone phosphate.
- **Amino Acids**: Glucogenic amino acids, such as alanine and glutamine, are deaminated to form intermediates like pyruvate and α-ketoglutarate, which enter the gluconeogenic pathway.
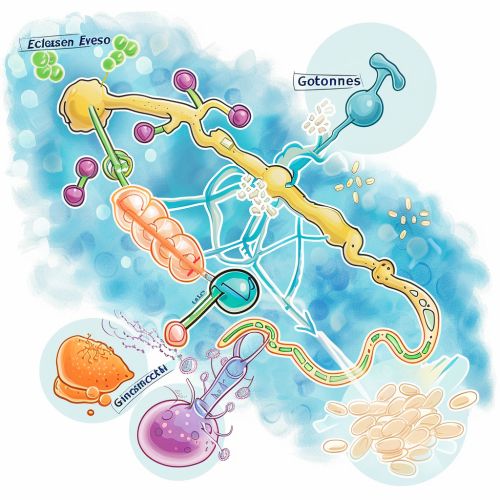
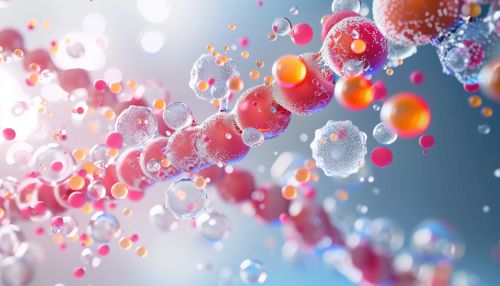
Regulation of Gluconeogenesis
The regulation of gluconeogenesis is complex and involves multiple layers of control, including allosteric regulation, covalent modification, and changes in gene expression. The interplay between insulin and glucagon is particularly crucial in this context.
Hormonal Regulation
- **Insulin**: Insulin inhibits gluconeogenesis by decreasing the transcription of key gluconeogenic enzymes such as PEPCK and glucose-6-phosphatase. It also promotes the phosphorylation (and inactivation) of fructose-1,6-bisphosphatase.
- **Glucagon**: In contrast, glucagon stimulates gluconeogenesis by increasing the levels of cyclic AMP (cAMP), which activates protein kinase A (PKA). PKA phosphorylates and activates enzymes involved in gluconeogenesis while inhibiting glycolytic enzymes.
Allosteric Regulation
- **Acetyl-CoA**: High levels of acetyl-CoA activate pyruvate carboxylase, promoting gluconeogenesis.
- **AMP**: Elevated AMP levels inhibit fructose-1,6-bisphosphatase, thereby downregulating gluconeogenesis.
Transcriptional Regulation
- **CREB**: The cAMP response element-binding protein (CREB) is activated by glucagon and promotes the transcription of gluconeogenic genes.
- **FOXO1**: Forkhead box protein O1 (FOXO1) is another transcription factor that enhances the expression of gluconeogenic enzymes. Insulin signaling inhibits FOXO1 by promoting its phosphorylation and subsequent degradation.
Physiological Significance
Gluconeogenesis is vital for maintaining blood glucose levels during periods when dietary glucose is not available. This is particularly important for tissues that rely heavily on glucose, such as the brain and red blood cells.
Fasting and Starvation
During fasting, glycogen stores are depleted within 24-48 hours, making gluconeogenesis the primary source of glucose. The liver increases gluconeogenic activity to supply glucose to peripheral tissues.
Exercise
Intense exercise leads to the production of lactate in muscles, which is transported to the liver and converted to glucose via the Cori cycle. This process helps to sustain prolonged physical activity by replenishing muscle glycogen stores.
Pathological Conditions
- **Diabetes Mellitus**: In diabetes, excessive gluconeogenesis contributes to hyperglycemia. Insulin resistance or deficiency fails to suppress hepatic gluconeogenesis, exacerbating the condition.
- **Alcoholic Ketoacidosis**: Chronic alcohol consumption can impair gluconeogenesis by depleting NAD+ levels, leading to hypoglycemia and ketoacidosis.
Clinical Implications
Understanding the regulation and function of gluconeogenesis has significant clinical implications, particularly in the management of metabolic disorders.
Therapeutic Targets
- **Metformin**: This antidiabetic drug inhibits hepatic gluconeogenesis by activating AMP-activated protein kinase (AMPK), which downregulates gluconeogenic genes.
- **Fasting Mimicking Diets**: These diets aim to reduce gluconeogenesis and improve metabolic health by mimicking the effects of fasting without severe caloric restriction.
Diagnostic Markers
- **Blood Glucose Levels**: Monitoring fasting blood glucose levels can provide insights into gluconeogenic activity.
- **Lactate and Pyruvate Ratios**: Abnormal lactate-to-pyruvate ratios can indicate dysregulation in gluconeogenesis, often seen in mitochondrial disorders.