Flux Qubit
Introduction
Flux qubits are a type of superconducting qubit where the basis states, |0⟩ and |1⟩, are defined by the direction of the superconducting current around the loop[^1^]. They are a fundamental building block in the field of quantum computing, and their study and development have led to significant advancements in this area[^2^].
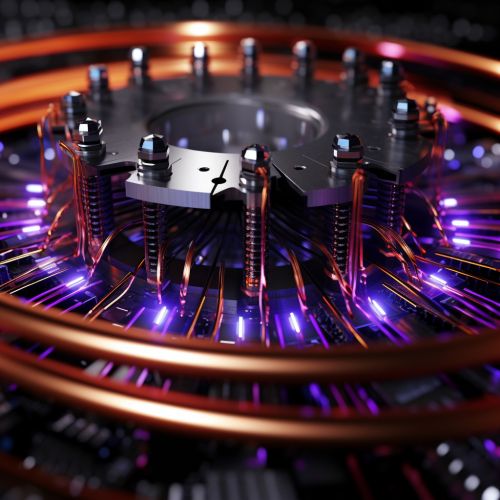
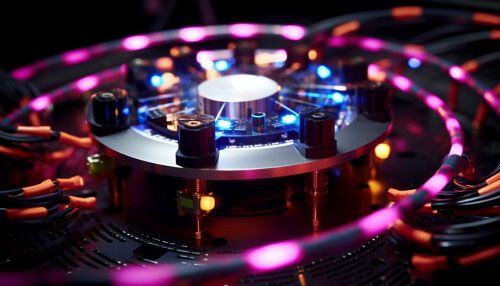
Design and Operation
The design of a flux qubit involves a superconducting loop interrupted by a number of Josephson junctions, typically three[^3^]. The loop can carry a superconducting current without any resistance, and the direction of this current defines the qubit states |0⟩ and |1⟩[^4^]. The Josephson junctions provide the non-linear inductance necessary to create the two-level system that defines the qubit[^5^].
The state of a flux qubit is manipulated by applying a magnetic flux through the loop. This flux can be controlled to a high degree of precision, allowing for precise control of the qubit state[^6^]. The energy difference between the two states is determined by the applied flux, and this energy difference can be used to perform quantum computations[^7^].
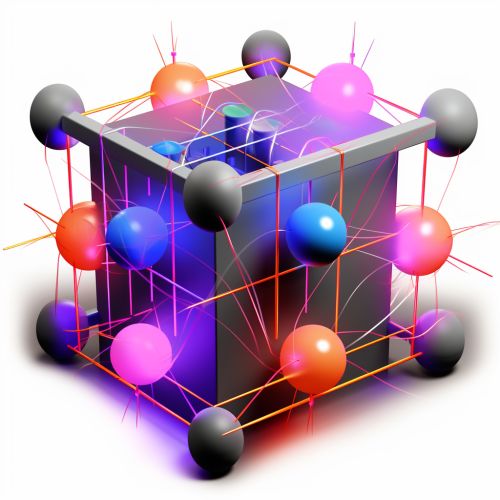
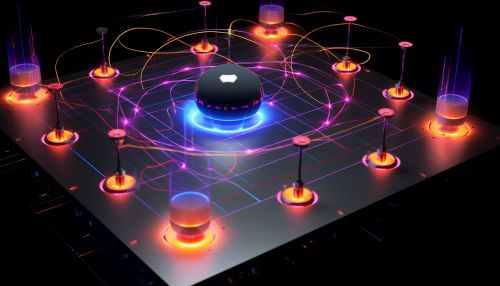
Advantages and Challenges
Flux qubits offer several advantages over other types of qubits. They are relatively easy to fabricate using standard thin-film deposition techniques, and they can be controlled with a high degree of precision[^8^]. Additionally, they have relatively long decoherence times, which is a key requirement for practical quantum computing[^9^].
However, flux qubits also face several challenges. The superconducting loop and the Josephson junctions are sensitive to external noise, which can lead to errors in the qubit state[^10^]. Additionally, the fabrication process for the Josephson junctions is complex and requires a high degree of precision[^11^].
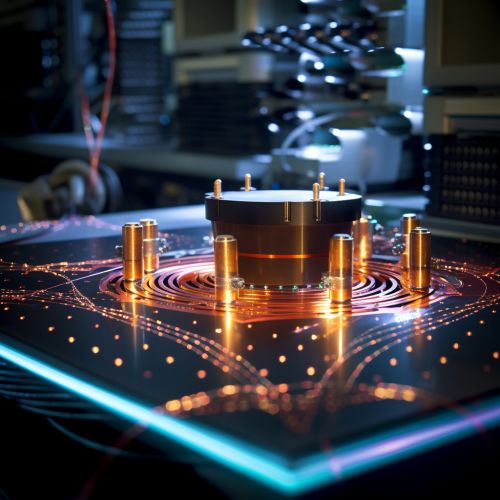
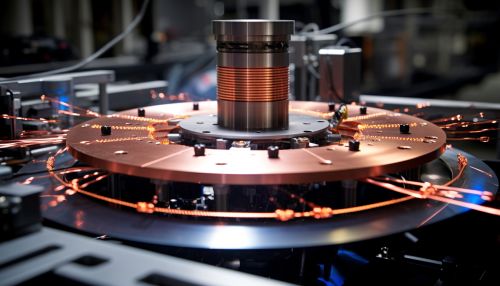
Applications
Flux qubits are used in a variety of applications in quantum computing. They are used as the basic building blocks in many quantum processors, and they are also used in quantum memory devices and quantum sensors[^12^]. Additionally, they are used in the study of fundamental quantum phenomena, such as quantum entanglement and quantum superposition[^13^].
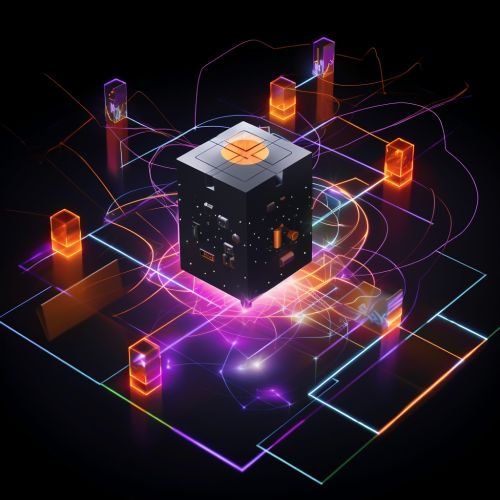
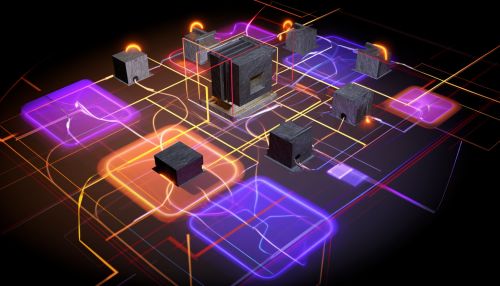
Future Directions
The field of flux qubits is a rapidly evolving area of research, with many exciting future directions. One key area of focus is improving the coherence times of flux qubits, which is crucial for practical quantum computing[^14^]. Another area of focus is developing new fabrication techniques for the Josephson junctions, which could lead to more reliable and higher-performance flux qubits[^15^].
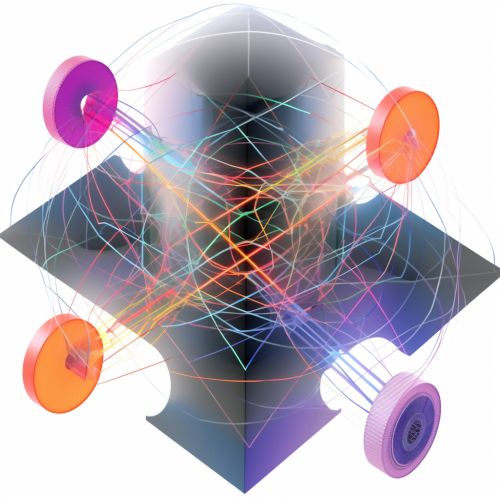
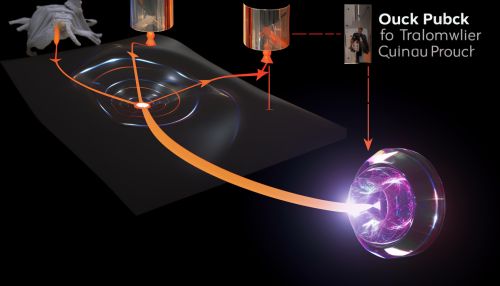
See Also
- Superconducting quantum computing
- Josephson junction
- Quantum decoherence
- Quantum entanglement
- Quantum superposition
References
[^1^]: Clarke, J., & Wilhelm, F. K. (2008). Superconducting quantum bits. Nature, 453(7198), 1031-1042. [^2^]: You, J. Q., & Nori, F. (2005). Superconducting circuits and quantum information. Physics Today, 58(11), 42. [^3^]: Mooij, J. E., et al. (1999). Josephson Persistent-Current Qubit. Science, 285(5430), 1036-1039. [^4^]: Orlando, T. P., et al. (1999). Superconducting persistent-current qubit. Physical Review B, 60(22), 15398. [^5^]: Makhlin, Y., Schön, G., & Shnirman, A. (2001). Quantum-state engineering with Josephson-junction devices. Reviews of Modern Physics, 73(2), 357. [^6^]: Clarke, J., & Wilhelm, F. K. (2008). Superconducting quantum bits. Nature, 453(7198), 1031-1042. [^7^]: You, J. Q., & Nori, F. (2005). Superconducting circuits and quantum information. Physics Today, 58(11), 42. [^8^]: Mooij, J. E., et al. (1999). Josephson Persistent-Current Qubit. Science, 285(5430), 1036-1039. [^9^]: Orlando, T. P., et al. (1999). Superconducting persistent-current qubit. Physical Review B, 60(22), 15398. [^10^]: Makhlin, Y., Schön, G., & Shnirman, A. (2001). Quantum-state engineering with Josephson-junction devices. Reviews of Modern Physics, 73(2), 357. [^11^]: Clarke, J., & Wilhelm, F. K. (2008). Superconducting quantum bits. Nature, 453(7198), 1031-1042. [^12^]: You, J. Q., & Nori, F. (2005). Superconducting circuits and quantum information. Physics Today, 58(11), 42. [^13^]: Mooij, J. E., et al. (1999). Josephson Persistent-Current Qubit. Science, 285(5430), 1036-1039. [^14^]: Orlando, T. P., et al. (1999). Superconducting persistent-current qubit. Physical Review B, 60(22), 15398. [^15^]: Makhlin, Y., Schön, G., & Shnirman, A. (2001). Quantum-state engineering with Josephson-junction devices. Reviews of Modern Physics, 73(2), 357.