Event Horizon
Introduction
An event horizon is a boundary in spacetime beyond which events cannot affect an outside observer. In layman's terms, it is defined as "the point of no return," i.e., the point at which the gravitational pull becomes so great as to make escape impossible, even for light. An event horizon is most commonly associated with black holes. Light emitted from beyond the event horizon can never reach the outside observer. Likewise, any object approaching the horizon from the observer's side appears to slow down and never quite pass through the horizon, with its image becoming more and more redshifted as time elapses.
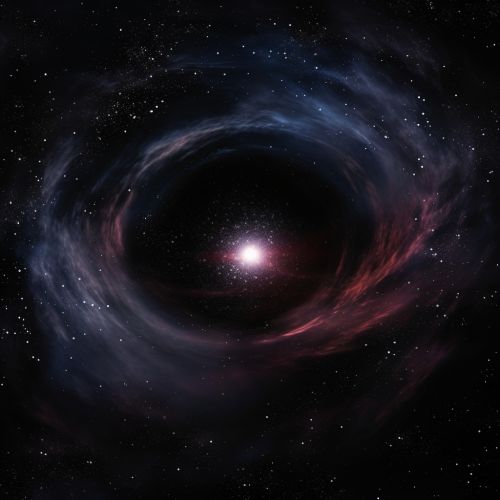
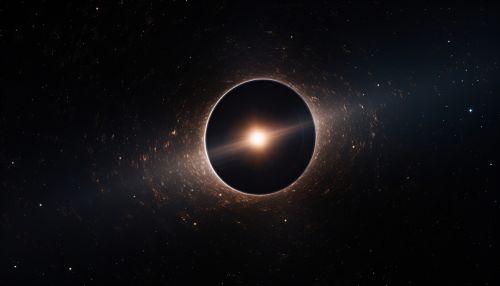
Theoretical Background
The concept of an event horizon was first proposed in the context of general relativity. According to general relativity, the gravitational field of a massive object alters the geometry of spacetime around it. As a result, the paths that light can take are bent towards the object. At the event horizon of a black hole, this bending becomes so intense that there are no paths that lead away from the black hole.
The event horizon of a black hole is linked to the object's escape velocity - the minimum speed needed for an object to escape from the gravitational influence of a massive body. At the event horizon, the escape velocity equals the speed of light. Since no object can reach or exceed the speed of light, no object can escape from inside the event horizon.
Types of Event Horizons
There are several types of event horizons, formed by different kinds of black holes and other phenomena. The most common types are associated with black holes, and they include:
1. Schwarzschild event horizons: These are associated with non-rotating, uncharged black holes, also known as Schwarzschild black holes. The event horizon is a spherical surface centered on the black hole.
2. Reissner-Nordström event horizons: These are associated with charged, non-rotating black holes, also known as Reissner-Nordström black holes. Depending on the charge of the black hole, it can have either one or two event horizons.
3. Kerr event horizons: These are associated with rotating, uncharged black holes, also known as Kerr black holes. The rotation causes the event horizon to be oblate, or squashed at the poles.
4. Kerr-Newman event horizons: These are associated with rotating, charged black holes, also known as Kerr-Newman black holes. The rotation and charge together cause the event horizon to have a complex shape.
5. Cosmological event horizons: These are associated with the expansion of the universe. They represent the boundary beyond which events cannot ever be observed.
Observational Evidence
While it is impossible to directly observe an event horizon, there are several pieces of observational evidence for their existence. These include:
1. Accretion disks: These are disks of matter that form around black holes. As matter falls towards the black hole, it heats up and emits X-rays, which can be detected by telescopes. The presence of an accretion disk suggests that there is a black hole at the center.
2. Gravitational lensing: This is the bending of light by gravity. When a massive object like a black hole passes in front of a distant light source, it can cause the light to bend and form a ring. This effect, known as an Einstein ring, is a prediction of general relativity and has been observed many times.
3. Gravitational waves: These are ripples in spacetime caused by the acceleration of massive objects. The detection of gravitational waves by the LIGO and VIRGO observatories in 2015 provided strong evidence for the existence of black holes and, by extension, event horizons.
Implications for Physics
The existence of event horizons has profound implications for physics, particularly in the realms of general relativity and quantum mechanics. The study of event horizons and black holes has led to the discovery of Hawking radiation, a theoretical prediction that black holes should emit radiation due to quantum effects near the event horizon. This has important implications for the fate of black holes and the information paradox.
Event horizons also have implications for the nature of spacetime itself. According to the cosmic censorship hypothesis, singularities - points where the curvature of spacetime becomes infinite - are always hidden behind an event horizon. This would mean that the "naked" singularities predicted by some solutions of the equations of general relativity do not actually exist in nature.