Electrochemical Water Splitting
Introduction
Electrochemical water splitting is a process that involves the decomposition of water into oxygen and hydrogen gas through the application of an electrical current. This process is fundamental to the production of Hydrogen as a clean and sustainable energy carrier. Water splitting is a critical component of Electrolysis, which is employed in various industrial applications, including the production of chemical feedstocks and energy storage systems. This article delves into the mechanisms, materials, and technological advancements associated with electrochemical water splitting.
Mechanisms of Water Splitting
Electrochemical water splitting consists of two half-reactions: the Oxygen Evolution Reaction (OER) and the Hydrogen Evolution Reaction (HER). These reactions occur at the anode and cathode, respectively, in an electrolytic cell.
Oxygen Evolution Reaction (OER)
The OER is a four-electron process that involves the oxidation of water molecules to produce oxygen gas. The reaction can be represented as: \[ 2H_2O(l) \rightarrow O_2(g) + 4H^+(aq) + 4e^- \]
The OER is often the rate-limiting step in water splitting due to its complex multi-electron transfer mechanism. Catalysts such as Iridium Oxide and Ruthenium Oxide are commonly used to enhance the efficiency of this reaction.
Hydrogen Evolution Reaction (HER)
The HER involves the reduction of protons to form hydrogen gas. The reaction is represented as: \[ 2H^+(aq) + 2e^- \rightarrow H_2(g) \]
Catalysts like Platinum are highly effective for the HER due to their excellent conductivity and catalytic properties. However, the high cost of platinum necessitates the exploration of alternative materials.
Materials for Electrochemical Water Splitting
The choice of materials for electrodes and catalysts is crucial for the efficiency and cost-effectiveness of electrochemical water splitting.
Electrode Materials
Electrodes must possess high electrical conductivity, chemical stability, and catalytic activity. Common materials include Graphite, Nickel, and Stainless Steel. Recent advancements have focused on developing Nanostructured Materials to enhance surface area and catalytic activity.
Catalyst Materials
Catalysts play a pivotal role in lowering the activation energy of the OER and HER. Transition metal oxides, phosphides, and sulfides are extensively researched as cost-effective alternatives to noble metal catalysts. Cobalt Oxide and Molybdenum Disulfide are examples of promising catalysts for water splitting.
Technological Advancements
Recent technological advancements have focused on improving the efficiency, durability, and scalability of electrochemical water splitting systems.
Photoelectrochemical Water Splitting
Photoelectrochemical Cells utilize solar energy to drive water splitting, integrating photovoltaic and electrochemical processes. Materials such as Titanium Dioxide and Bismuth Vanadate are explored for their photoactive properties.
Solid Oxide Electrolysis Cells (SOECs)
SOECs operate at high temperatures, utilizing solid oxide electrolytes to achieve high efficiency in water splitting. These systems are particularly suitable for integration with Renewable Energy sources.
Proton Exchange Membrane Electrolysis
Proton exchange membrane (PEM) electrolysis employs a solid polymer electrolyte to facilitate ion transport. PEM electrolyzers are known for their compact design and rapid response to fluctuating power inputs, making them ideal for Grid Integration.
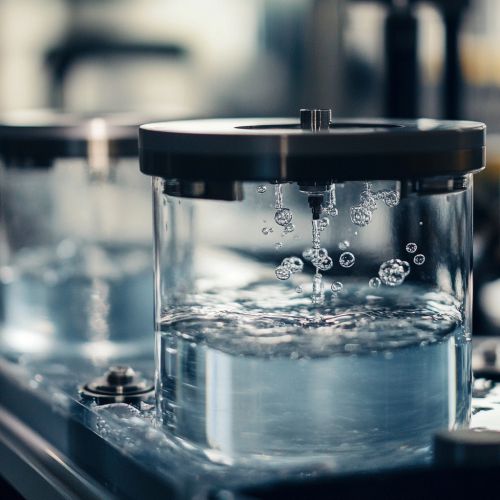
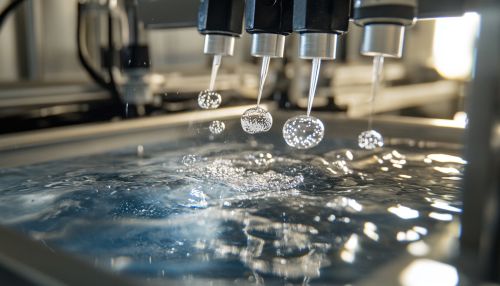
Challenges and Future Directions
Despite significant progress, several challenges remain in the widespread adoption of electrochemical water splitting.
Cost and Material Availability
The high cost and limited availability of certain catalyst materials, such as platinum and iridium, pose economic challenges. Research is ongoing to develop earth-abundant and low-cost alternatives.
Efficiency and Durability
Improving the efficiency and durability of water splitting systems is critical for commercial viability. Strategies include optimizing catalyst composition and electrode design to enhance performance and longevity.
Integration with Renewable Energy
Integrating water splitting systems with intermittent renewable energy sources requires advancements in energy storage and system flexibility. Hybrid systems combining electrolysis with Battery Storage or Fuel Cells are being explored.
Environmental and Economic Implications
Electrochemical water splitting holds potential for reducing carbon emissions and promoting energy independence. The production of hydrogen from water using renewable energy sources can significantly decrease reliance on fossil fuels.
Environmental Impact
The environmental benefits of water splitting are contingent on the use of renewable energy. The lifecycle assessment of electrolyzers, including material extraction and disposal, is crucial for evaluating their sustainability.
Economic Viability
The economic feasibility of electrochemical water splitting depends on factors such as electricity cost, system efficiency, and hydrogen market demand. Government policies and incentives play a vital role in fostering the growth of hydrogen technologies.