Dual-chamber microbial fuel cell
Introduction
A MFC is a bio-electrochemical system that drives an electric current by using bacteria and mimicking bacterial interactions found in nature. MFCs can be grouped into two general categories: those that use a mediator and those that are mediator-less. The first category includes MFCs that use a mediator to transfer electrons produced by the bacteria to the electrode. In the second category, the bacteria transfer electrons directly to the electrode. A dual-chamber microbial fuel cell (D-MFC) is a type of MFC where the anode and cathode compartments are separated by a proton exchange membrane.
Working Principle
The working principle of a D-MFC involves several steps. First, the bacteria in the anode compartment oxidize the organic matter. This process releases electrons and protons, and produces carbon dioxide. The electrons are transferred to the anode, and then move through an external circuit to the cathode. The protons move through the proton exchange membrane to the cathode compartment. In the cathode compartment, oxygen (from air) and protons react with the electrons to form water. The movement of electrons from the anode to the cathode generates an electric current.
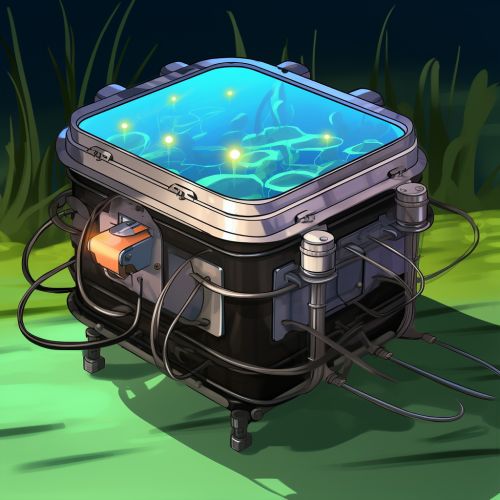
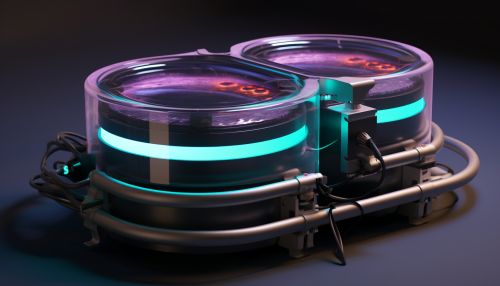
Components
A D-MFC consists of an anode compartment, a cathode compartment, a proton exchange membrane, an external circuit, and a load. The anode and cathode compartments are usually made of a material that is non-conductive to electricity, such as plastic or glass. The anode and cathode are made of a conductive material, such as graphite or metal, and are coated with a layer of bacteria. The proton exchange membrane is usually made of a material like Nafion, which is permeable to protons but not to electrons. The external circuit connects the anode and cathode, and the load is the device that uses the electricity generated by the MFC.
Applications
D-MFCs have a wide range of potential applications. They can be used for wastewater treatment, biosensors, biohydrogen production, and energy production. In wastewater treatment, D-MFCs can degrade organic matter in the wastewater, thus reducing the need for aeration and the production of sludge. As biosensors, D-MFCs can detect the presence of certain chemicals in the environment, such as heavy metals or pollutants. In biohydrogen production, D-MFCs can produce hydrogen gas as a byproduct of the oxidation of organic matter. In energy production, D-MFCs can generate electricity from organic waste, thus providing a renewable source of energy.
Advantages and Disadvantages
The main advantage of D-MFCs is that they can generate electricity from organic waste, which is a renewable resource. They can also operate at room temperature and atmospheric pressure, which makes them suitable for use in a wide range of environments. However, D-MFCs also have some disadvantages. The power output of D-MFCs is relatively low compared to other types of fuel cells. The performance of D-MFCs can also be affected by factors such as the type of bacteria used, the type of substrate, and the operating conditions.
Research and Development
Research and development in the field of D-MFCs is focused on improving their performance and finding new applications. Some of the research topics include the optimization of the microbial community, the development of new materials for the anode and cathode, the improvement of the proton exchange membrane, and the increase of the power density. There is also interest in integrating D-MFCs with other technologies, such as solar cells or wind turbines, to create hybrid systems that can provide a more stable and reliable source of energy.