Decellularized Extracellular Matrix
Introduction
The decellularized extracellular matrix (dECM) is a type of biomaterial that has been stripped of all cellular components, leaving behind a complex network of proteins and sugars that provide structural and biochemical support to cells. This matrix is a critical component of tissue engineering and regenerative medicine, as it provides a natural scaffold for cell growth and differentiation.
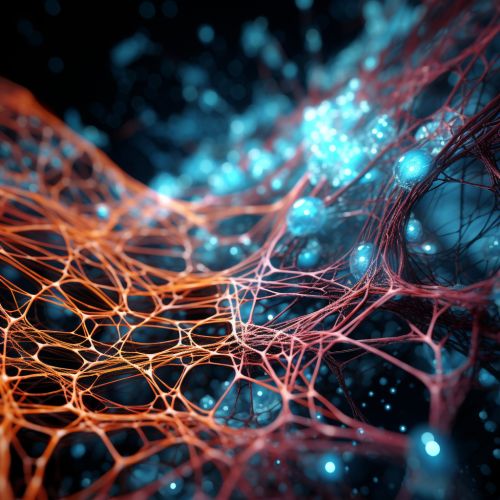
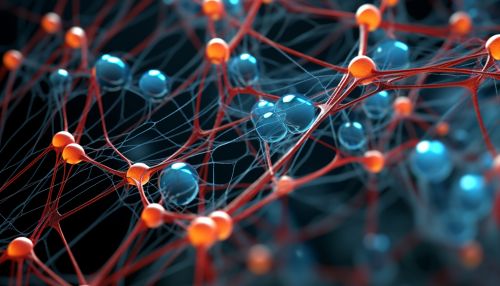
Structure and Composition
The extracellular matrix (ECM) is composed of a variety of proteins and sugars that provide structural support to cells. These include collagen, the most abundant protein in the ECM, elastin, which provides elasticity, and fibronectin, which aids in cell adhesion. Additionally, the ECM contains a variety of glycosaminoglycans (GAGs), which are long, unbranched polysaccharides that provide hydration and resistance to compression.
Decellularization Process
The process of decellularization involves removing all cellular components from a tissue or organ, leaving behind the ECM. This is typically achieved through a combination of physical, chemical, and enzymatic methods. Physical methods include freezing and thawing, which disrupts cell membranes, and agitation, which helps to dislodge cells. Chemical methods often involve detergents, which solubilize cell membranes, and acids or bases, which can break down nuclear material. Enzymatic methods typically involve DNases and RNases, which degrade DNA and RNA, respectively.
Applications in Tissue Engineering and Regenerative Medicine
dECM has a wide range of applications in tissue engineering and regenerative medicine. It can be used as a scaffold for cell growth and differentiation, providing a natural environment that mimics the in vivo conditions. This can be particularly useful in the engineering of complex tissues and organs, such as the heart, liver, and lungs.
In addition to providing a structural scaffold, dECM also provides biochemical cues that can guide cell behavior. For example, the presence of specific proteins in the ECM can influence cell adhesion, migration, proliferation, and differentiation. Furthermore, the mechanical properties of the ECM, such as its stiffness and elasticity, can also influence cell behavior.
Challenges and Future Directions
While dECM holds great promise for tissue engineering and regenerative medicine, there are also several challenges that need to be addressed. One of the main challenges is the potential for immune response. While the decellularization process removes cellular antigens that can trigger an immune response, some residual cellular material may remain, which could potentially trigger an immune response.
Another challenge is the preservation and storage of dECM. While fresh dECM can be used immediately, it is often necessary to store dECM for later use. However, current preservation methods, such as freezing and lyophilization, can alter the structure and composition of the ECM, potentially affecting its performance.
Despite these challenges, the field of dECM research is rapidly advancing, and new methods are being developed to improve the decellularization process, reduce immune response, and preserve the ECM. With continued research and development, dECM has the potential to revolutionize the field of tissue engineering and regenerative medicine.