Dark Matter Detection in Astrophysics
Introduction
Dark matter is a form of matter that is thought to account for approximately 85% of the matter in the universe and about a quarter of its total energy density. The majority of dark matter is believed to be non-baryonic in nature, possibly being composed of some as-yet undiscovered subatomic particles. Its presence is implied in a variety of astrophysical observations, including gravitational effects which cannot be explained by accepted theories of gravity unless more matter is present than can be seen. For this reason, most experts think dark matter to be abundant in the universe and to have had a strong influence on its structure and evolution. Dark matter is called dark because it does not appear to interact with observable electromagnetic radiation, such as light, and is thus invisible to the entire electromagnetic spectrum, making it extremely difficult to detect.
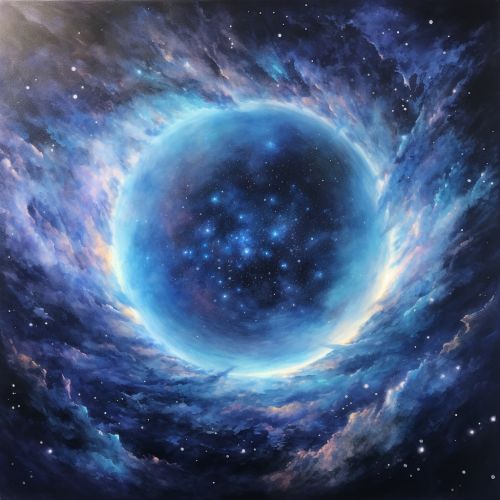
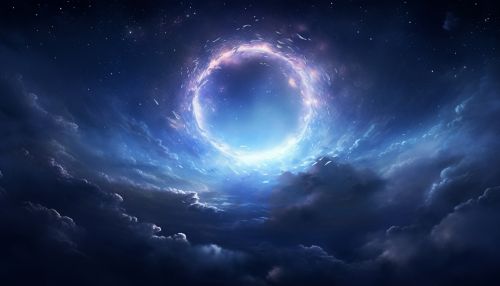
Detection Methods
The search for dark matter involves two complementary approaches: direct detection and indirect detection.
Direct Detection
Direct detection experiments aim to observe low-energy recoils (typically a few keVs) of atomic nuclei induced by interactions with particles of dark matter, often in the form of WIMPs. These experiments are performed in deep underground laboratories to reduce the background noise from cosmic rays. The detectors are typically composed of a material with a high atomic number and low energy threshold. They are designed to measure the direction, energy, and timing of the recoiling nuclei.
Indirect Detection
Indirect detection involves looking for the byproducts of dark matter particle annihilations or decays. These byproducts could take the form of standard model particles such as photons, neutrinos, or cosmic rays. Observations of the cosmic microwave background radiation (CMBR) and large scale structure of the universe can also provide indirect evidence of dark matter.
Current Experiments
There are numerous ongoing experiments aiming to detect dark matter particles. These include the Large Underground Xenon (LUX) experiment, the Cryogenic Dark Matter Search (CDMS), the XENON1T experiment, and the China Jinping Underground Laboratory's PandaX experiment.
LUX Experiment
The LUX experiment is a direct detection experiment that uses liquid xenon as the target material. It is located in the Sanford Underground Research Facility in South Dakota, USA.
CDMS Experiment
The CDMS experiment is a series of experiments that use cryogenically cooled detectors made of germanium and silicon in an attempt to detect dark matter particles.
XENON1T Experiment
The XENON1T experiment is a direct detection experiment that uses a dual-phase xenon time projection chamber. It is located at the Gran Sasso National Laboratory in Italy.
PandaX Experiment
The PandaX experiment is a direct detection experiment that uses a dual-phase xenon time projection chamber. It is located at the China Jinping Underground Laboratory in Sichuan, China.
Challenges in Dark Matter Detection
Despite the numerous ongoing experiments, dark matter detection remains a significant challenge. This is due to several reasons. First, the interactions of dark matter particles with normal matter are expected to be very weak, making them difficult to detect. Second, there is a significant background noise from other particles, such as neutrinos, which can mimic the signals expected from dark matter particles. Third, the exact properties of dark matter particles, such as their mass and interaction cross-section, are still unknown, making it difficult to design experiments to detect them.
Future Prospects
Despite the challenges, the search for dark matter continues to be a major focus of astrophysical research. Future experiments will continue to push the boundaries of sensitivity and will explore a wider range of dark matter particle properties. These include the next generation of direct detection experiments, such as the LUX-ZEPLIN (LZ) experiment and the XENONnT experiment, as well as indirect detection experiments such as the Fermi Gamma-ray Space Telescope and the IceCube Neutrino Observatory.