Bone Tissue Engineering
Introduction
Bone tissue engineering is a rapidly advancing field within the broader discipline of regenerative medicine. The primary goal of bone tissue engineering is to develop biological substitutes that can restore, maintain, or improve the function of damaged bone tissue. This is achieved through the combination of biomaterials, stem cells, and growth factors to create a construct that can mimic the natural bone environment and promote bone regeneration.
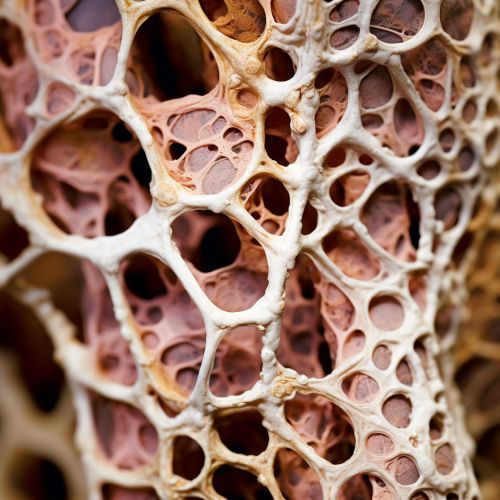
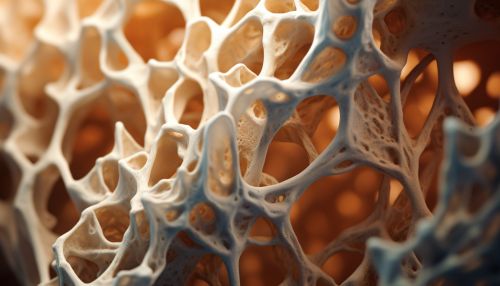
Biomaterials in Bone Tissue Engineering
Biomaterials play a crucial role in bone tissue engineering. They provide a three-dimensional (3D) structure or scaffold that supports cell attachment, proliferation, and differentiation. The ideal biomaterial for bone tissue engineering should be biocompatible, biodegradable, osteoconductive, osteoinductive, and have mechanical properties similar to those of the natural bone.
There are various types of biomaterials used in bone tissue engineering, including natural and synthetic polymers, ceramics, and composites. Natural polymers such as collagen and gelatin have excellent biocompatibility and bioactivity but lack mechanical strength. Synthetic polymers like poly(lactic-co-glycolic acid) (PLGA) and poly(caprolactone) (PCL) have tunable mechanical properties and degradation rates but may lack bioactivity. Ceramics such as hydroxyapatite and tricalcium phosphate are highly osteoconductive and have similar mineral composition to the natural bone but are brittle. Composites that combine different types of biomaterials are often used to overcome the limitations of individual materials.
Stem Cells in Bone Tissue Engineering
Stem cells are another critical component in bone tissue engineering. They have the ability to self-renew and differentiate into various cell types, including osteoblasts, the cells responsible for bone formation. There are several sources of stem cells used in bone tissue engineering, including bone marrow-derived mesenchymal stem cells (BMSCs), adipose-derived stem cells (ADSCs), and induced pluripotent stem cells (iPSCs).
BMSCs are the most commonly used stem cells in bone tissue engineering due to their high osteogenic potential. However, their isolation requires an invasive procedure and their number and differentiation potential decrease with age. ADSCs are an attractive alternative as they can be obtained in large quantities through a less invasive procedure and have similar osteogenic potential to BMSCs. iPSCs can be generated from adult somatic cells and have unlimited self-renewal capacity and pluripotency, but their use is associated with ethical concerns and potential risks of tumorigenicity.
Growth Factors in Bone Tissue Engineering
Growth factors are proteins that regulate cell behavior, including proliferation, differentiation, and migration. In bone tissue engineering, they are used to stimulate the osteogenic differentiation of stem cells and promote bone regeneration. The most commonly used growth factors in bone tissue engineering are bone morphogenetic proteins (BMPs), particularly BMP-2 and BMP-7, which have strong osteoinductive properties. However, their use is associated with potential side effects, including ectopic bone formation and inflammation, and high costs.
Other growth factors used in bone tissue engineering include transforming growth factor-beta (TGF-β), fibroblast growth factors (FGFs), and vascular endothelial growth factors (VEGFs). TGF-β enhances the proliferation of stem cells and their production of extracellular matrix, FGFs stimulate angiogenesis and osteoblast differentiation, and VEGFs promote vascularization, which is crucial for the survival of the engineered bone tissue.
Challenges and Future Directions
Despite significant advancements, there are still several challenges to be addressed in bone tissue engineering. One of the main challenges is the vascularization of the engineered bone tissue. Without a sufficient blood supply, the cells in the interior of the tissue scaffold may die, leading to the failure of the engineered bone tissue. Strategies to enhance the vascularization of the engineered bone tissue include the use of angiogenic growth factors, co-culture of stem cells with endothelial cells, and the design of biomaterials with hierarchical porosity.
Another challenge is the scale-up of the engineered bone tissue for the treatment of large bone defects. Current tissue engineering approaches are limited to small-scale constructs due to the diffusion limits of nutrients and oxygen. Bioreactor technologies that can provide dynamic culture conditions and enhance the mass transfer of nutrients and oxygen are being developed to address this issue.
The integration of the engineered bone tissue with the host bone is also a challenge. Strategies to enhance the integration include the surface modification of the biomaterials to improve their bioactivity and the use of growth factors to stimulate the migration and differentiation of the host cells.
Future directions in bone tissue engineering include the development of smart biomaterials that can respond to the physiological environment and regulate cell behavior, the use of gene therapy to enhance the osteogenic potential of stem cells, and the application of nanotechnology to improve the delivery of growth factors and other bioactive molecules.