Antifreeze Protein
Introduction
Antifreeze proteins (AFPs), also known as ice structuring proteins (ISPs), are a class of polypeptides produced by certain vertebrates, plants, fungi, and bacteria that permit their survival in subzero environments. AFPs bind to small ice crystals to inhibit growth and recrystallization of ice that would otherwise be fatal.
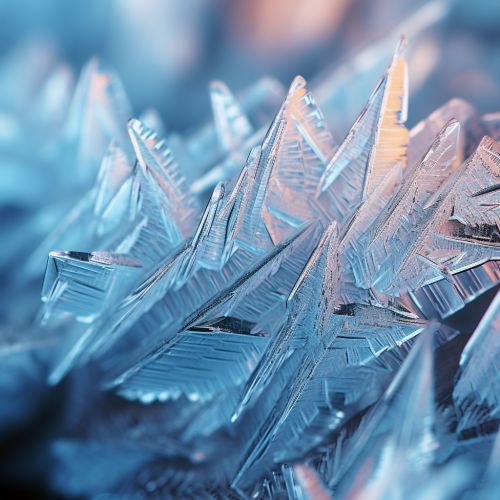
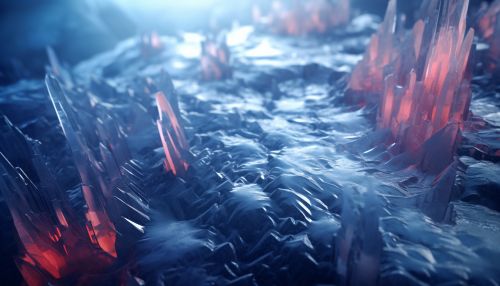
Structure and Function
Antifreeze proteins are characterized by their unique structure and function. They are typically composed of several repeating amino acid sequences, which fold into a specific three-dimensional structure. This structure allows the proteins to bind to the surface of ice crystals and inhibit their growth.
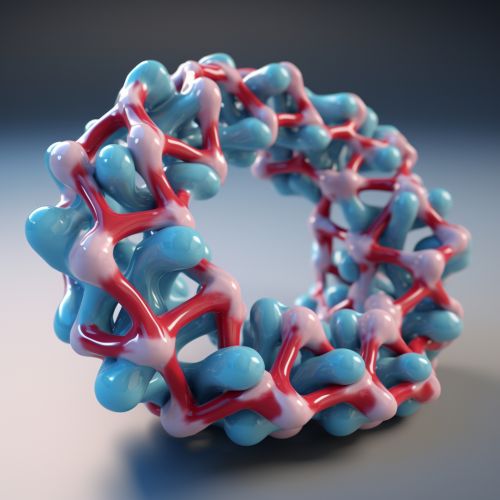
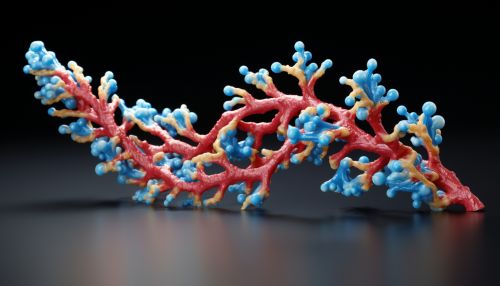
The primary function of AFPs is to lower the freezing temperature of water inside the organisms that produce them, preventing the water from freezing solid and damaging the organism's cells. This is achieved by a process known as 'thermal hysteresis' – a phenomenon where the freezing point of a solution is lower than its melting point. AFPs are able to lower the freezing point without affecting the melting point, resulting in a 'thermal hysteresis gap'[^1^].
Types of Antifreeze Proteins
There are several types of AFPs, each with a unique structure and function. These include:
- Type I AFPs: These are small, alanine-rich proteins found in fish such as the winter flounder and Arctic cod. They are typically composed of a single alpha-helix and are rich in alanine residues[^2^].
- Type II AFPs: These are larger, cysteine-rich proteins found in sea raven and smelt. They are characterized by their high cysteine content and the presence of disulfide bonds[^3^].
- Type III AFPs: These are globular proteins found in Antarctic notothenioid fish. They are composed of a single polypeptide chain that folds into a compact, globular structure[^4^].
- Antifreeze glycoproteins (AFGPs): These are found in Antarctic and Arctic fish. They are composed of a repeating unit of three amino acids and a disaccharide, which is believed to be responsible for their antifreeze activity[^5^].
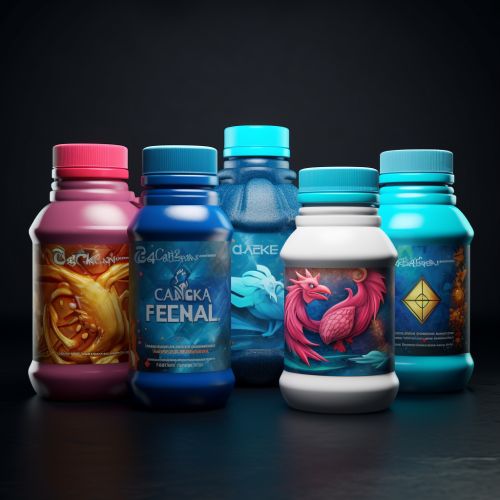
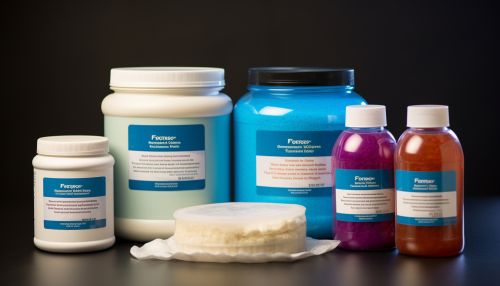
Mechanism of Action
The mechanism by which AFPs inhibit ice growth is complex and not fully understood. However, it is believed that AFPs bind to the surface of ice crystals and inhibit their growth by a process known as 'adsorption-inhibition'. In this process, AFPs adsorb onto the surface of ice crystals and prevent water molecules from joining the crystal lattice[^6^].
In addition to adsorption-inhibition, AFPs also inhibit ice recrystallization, a process where larger ice crystals grow at the expense of smaller ones. This is particularly important in the prevention of freeze-thaw damage, as recrystallization can lead to the formation of large ice crystals that can puncture cell membranes[^7^].
Biological Role and Significance
The primary role of AFPs is to protect the organisms that produce them from freezing. In cold environments, ice formation can lead to cell damage and death. By inhibiting ice growth and recrystallization, AFPs allow these organisms to survive in subzero temperatures.
In addition to their role in freeze protection, AFPs also have other biological roles. For example, in plants, AFPs have been shown to enhance cold tolerance, improve water use efficiency, and increase resistance to drought and salinity[^8^].
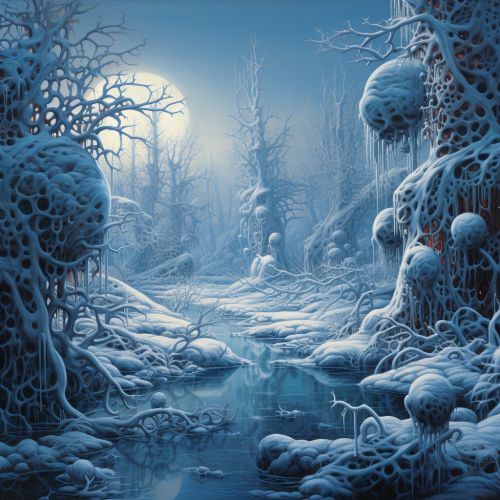
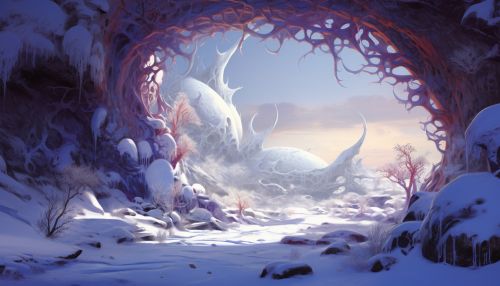
Applications
Due to their unique properties, AFPs have potential applications in a variety of fields. These include:
- Cryopreservation: AFPs could be used to improve the cryopreservation of biological samples, such as cells, tissues, and organs. By inhibiting ice growth and recrystallization, AFPs could reduce the damage caused by freezing and thawing[^9^].
- Food industry: AFPs could be used to improve the texture and quality of frozen foods. By inhibiting ice recrystallization, AFPs could prevent the formation of large ice crystals that can negatively affect the texture of frozen foods[^10^].
- Medical applications: AFPs could be used in the development of new treatments for conditions such as frostbite and hypothermia. They could also be used to improve the preservation of organs for transplantation[^11^].
See Also
References
[^1^]: Davies, P. L. (2014). Ice-binding proteins: a remarkable diversity of structures for stopping and starting ice growth. Trends in Biochemical Sciences, 39(11), 548-555. [^2^]: Cheng, C. H., & Detrich, H. W. (2007). Molecular ecophysiology of Antarctic notothenioid fishes. Philosophical Transactions of the Royal Society B: Biological Sciences, 362(1488), 2215-2232. [^3^]: Fletcher, G. L., Hew, C. L., & Davies, P. L. (2001). Antifreeze proteins of teleost fishes. Annual Review of Physiology, 63, 359-390. [^4^]: DeVries, A. L., & Cheng, C. H. (2005). Antifreeze proteins and organismal freezing avoidance in polar fishes. In Fish Physiology (Vol. 22, pp. 155-201). Academic Press. [^5^]: Ewart, K. V., & Fletcher, G. L. (1993). Heterogeneity in the antifreeze protein gene (afp) family of the Atlantic snailfish, Liparis atlanticus. Biochimica et Biophysica Acta (BBA)-Gene Structure and Expression, 1172(1-2), 83-92. [^6^]: Knight, C. A., Cheng, C. C., & DeVries, A. L. (1991). Adsorption of alpha-helical antifreeze peptides on specific ice crystal surface planes. Biophysical Journal, 59(2), 409-418. [^7^]: Graether, S. P., & Jia, Z. (2001). Modeling Pseudomonas syringae ice-nucleation protein as a beta-helical protein. Biophysical Journal, 80(3), 1169-1173. [^8^]: Wisniewski, M., Webb, R., Balsamo, R., Close, T. J., Yu, X. M., & Griffith, M. (1999). Purification, immunolocalization, cryoprotective, and antifreeze activity of PCA60: A dehydrin from peach (Prunus persica). Physiologia Plantarum, 105(4), 600-608. [^9^]: Rubinsky, B., Arav, A., & DeVries, A. L. (1992). The cryoprotective effect of antifreeze glycopeptides from antarctic fishes. Cryobiology, 29(1), 69-79. [^10^]: Goff, H. D., & Hill, A. R. (1991). Ice cream. US Patent No. 5,034,218. [^11^]: Rubinsky, B., & Pegg, D. E. (1988). A mathematical model for the freezing process in biological tissue. Proceedings of the Royal Society of London. Series B. Biological Sciences, 234(1277), 343-358.