Frequency Doubling: Difference between revisions
(Created page with "== Introduction == Frequency doubling, also known as second harmonic generation (SHG), is a nonlinear optical process in which photons interacting with a nonlinear material are effectively combined to form new photons with twice the energy, and therefore twice the frequency, of the original photons. This phenomenon is a fundamental aspect of nonlinear optics and has significant applications in various fields, including laser technology, telecommunications, and medical i...") |
No edit summary |
||
Line 23: | Line 23: | ||
Medical imaging also benefits from frequency doubling, particularly in techniques such as multiphoton microscopy. This imaging modality uses frequency-doubled light to excite fluorescent dyes in biological tissues, providing high-resolution images with minimal photodamage. | Medical imaging also benefits from frequency doubling, particularly in techniques such as multiphoton microscopy. This imaging modality uses frequency-doubled light to excite fluorescent dyes in biological tissues, providing high-resolution images with minimal photodamage. | ||
[[Image:Detail-104457.jpg|thumb|center|Close-up of a laser beam passing through a nonlinear crystal, emitting green light.]] | |||
== Theoretical Framework == | == Theoretical Framework == |
Revision as of 10:48, 22 November 2024
Introduction
Frequency doubling, also known as second harmonic generation (SHG), is a nonlinear optical process in which photons interacting with a nonlinear material are effectively combined to form new photons with twice the energy, and therefore twice the frequency, of the original photons. This phenomenon is a fundamental aspect of nonlinear optics and has significant applications in various fields, including laser technology, telecommunications, and medical imaging.
Principles of Frequency Doubling
Frequency doubling occurs when a material with a non-centrosymmetric crystal structure is exposed to intense electromagnetic fields, such as those produced by a laser. The nonlinear interaction between the electromagnetic field and the material results in the generation of new photons at twice the frequency of the incident photons. This process can be mathematically described by the nonlinear polarization of the medium, which is expressed as a power series in the electric field. The second-order term in this series is responsible for second harmonic generation.
The efficiency of frequency doubling depends on several factors, including the intensity of the incident light, the phase matching conditions, and the properties of the nonlinear material. Phase matching is a critical condition that ensures the conservation of momentum and maximizes the conversion efficiency. It involves aligning the propagation vectors of the fundamental and second harmonic waves so that they remain in phase as they travel through the medium.
Nonlinear Optical Materials
The choice of material is crucial for efficient frequency doubling. Nonlinear optical materials must possess a high second-order nonlinear susceptibility and a non-centrosymmetric crystal structure. Common materials used for frequency doubling include beta barium borate (BBO), lithium niobate (LiNbO3), and potassium titanyl phosphate (KTP). Each of these materials has unique properties that make them suitable for specific applications.
Beta barium borate is known for its wide transparency range and high damage threshold, making it ideal for high-power laser applications. Lithium niobate, on the other hand, is valued for its high nonlinear coefficient and ease of fabrication. Potassium titanyl phosphate is often used in compact laser systems due to its high efficiency and thermal stability.
Applications of Frequency Doubling
Frequency doubling has a wide range of applications across various industries. In laser technology, it is commonly used to convert infrared laser light into visible light. For example, a Nd:YAG laser operating at 1064 nm can be frequency doubled to produce green light at 532 nm. This process is essential for applications requiring visible laser light, such as laser pointers, displays, and biomedical imaging.
In telecommunications, frequency doubling is used to generate blue and ultraviolet light for optical data storage and high-density optical communication systems. The ability to produce shorter wavelengths allows for higher data transmission rates and increased storage capacity.
Medical imaging also benefits from frequency doubling, particularly in techniques such as multiphoton microscopy. This imaging modality uses frequency-doubled light to excite fluorescent dyes in biological tissues, providing high-resolution images with minimal photodamage.
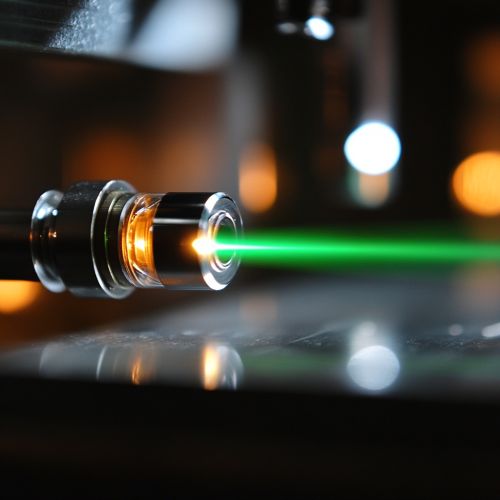
Theoretical Framework
The theoretical framework for frequency doubling is grounded in the principles of nonlinear optics. The process can be described using the coupled wave equations, which govern the interaction between the fundamental and second harmonic waves. These equations take into account the nonlinear polarization, phase matching conditions, and the depletion of the fundamental wave as energy is transferred to the second harmonic wave.
The efficiency of frequency doubling is often quantified by the conversion efficiency, defined as the ratio of the power of the second harmonic wave to the power of the fundamental wave. This efficiency is influenced by factors such as the coherence length, which is the distance over which the fundamental and second harmonic waves remain in phase, and the walk-off angle, which describes the angular separation between the two waves due to birefringence in the nonlinear material.
Advances in Frequency Doubling Technology
Recent advances in frequency doubling technology have focused on improving conversion efficiency and expanding the range of available wavelengths. One approach involves the use of quasi-phase matching (QPM), a technique that periodically reverses the sign of the nonlinear susceptibility in the material to achieve phase matching over a broader range of wavelengths. This method has been successfully implemented in periodically poled lithium niobate (PPLN) and periodically poled potassium titanyl phosphate (PPKTP).
Another area of research is the development of new nonlinear materials with enhanced properties. Organic nonlinear crystals, such as DAST (4-N,N-dimethylamino-4'-N'-methyl-stilbazolium tosylate), offer high nonlinear coefficients and broad transparency ranges, making them promising candidates for future frequency doubling applications.
Challenges and Limitations
Despite its many advantages, frequency doubling faces several challenges and limitations. One of the primary challenges is achieving efficient phase matching, particularly for short wavelengths in the ultraviolet region. The limited availability of suitable nonlinear materials for specific wavelength ranges also poses a challenge.
Thermal effects, such as heating and thermal lensing, can degrade the performance of frequency doubling systems, especially at high power levels. These effects can lead to a reduction in conversion efficiency and beam quality. Strategies to mitigate thermal effects include the use of temperature-controlled environments and the development of materials with high thermal conductivity.
Future Directions
The future of frequency doubling technology lies in the continued development of new materials and techniques to enhance efficiency and expand the range of accessible wavelengths. Advances in nanotechnology and material science hold promise for the creation of novel nonlinear materials with tailored properties.
Integration of frequency doubling systems into compact and portable devices is another area of interest, driven by the demand for miniaturized laser systems in applications such as portable medical diagnostics and consumer electronics.