Quantum Hypothesis: Difference between revisions
(Created page with "== Introduction == The quantum hypothesis is a fundamental concept in quantum mechanics, which proposes that energy is quantized and can exist only in discrete amounts, or "quanta." This hypothesis was first introduced by Max Planck in 1900 to explain black-body radiation and later expanded by Albert Einstein to explain the photoelectric effect. The quantum hypothesis laid the groundwork for the development of quantum theory, which has since become a corn...") |
No edit summary |
||
Line 62: | Line 62: | ||
* [[Quantum Cryptography]] | * [[Quantum Cryptography]] | ||
[[Image:Detail-92527.jpg|thumb|center|Photograph of a quantum computer with visible qubits and cooling system.|class=only_on_mobile]] | |||
[[Image:Detail-92528.jpg|thumb|center|Photograph of a quantum computer with visible qubits and cooling system.|class=only_on_desktop]] | |||
== References == | == References == |
Latest revision as of 22:07, 14 June 2024
Introduction
The quantum hypothesis is a fundamental concept in quantum mechanics, which proposes that energy is quantized and can exist only in discrete amounts, or "quanta." This hypothesis was first introduced by Max Planck in 1900 to explain black-body radiation and later expanded by Albert Einstein to explain the photoelectric effect. The quantum hypothesis laid the groundwork for the development of quantum theory, which has since become a cornerstone of modern physics.
Historical Background
The quantum hypothesis emerged in the early 20th century as scientists sought to resolve inconsistencies in classical physics. Classical theories, such as Newtonian mechanics and electromagnetism, could not explain certain phenomena observed at the atomic and subatomic levels. The failure of classical physics to account for the ultraviolet catastrophe in black-body radiation experiments led Max Planck to propose that energy is emitted or absorbed in discrete units called "quanta."
Planck's Contribution
Max Planck's work on black-body radiation marked the inception of the quantum hypothesis. In 1900, Planck introduced the idea that the energy of electromagnetic waves is quantized and can be described by the equation \( E = h \nu \), where \( E \) is the energy, \( h \) is Planck's constant, and \( \nu \) is the frequency of the radiation. This quantization of energy resolved the ultraviolet catastrophe and provided a new understanding of thermal radiation.
Einstein's Extension
Albert Einstein extended Planck's quantum hypothesis to explain the photoelectric effect in 1905. Einstein proposed that light consists of discrete packets of energy called photons, each with energy \( E = h \nu \). This explanation accounted for the observation that light can eject electrons from a metal surface only if its frequency exceeds a certain threshold, regardless of its intensity. Einstein's work on the photoelectric effect provided strong evidence for the quantum nature of light and earned him the Nobel Prize in Physics in 1921.
Development of Quantum Theory
The quantum hypothesis spurred the development of quantum theory, which describes the behavior of particles at the atomic and subatomic levels. Quantum theory encompasses several key principles, including wave-particle duality, the uncertainty principle, and quantum superposition.
Wave-Particle Duality
Wave-particle duality is the concept that particles, such as electrons and photons, exhibit both wave-like and particle-like properties. This duality was first demonstrated by the double-slit experiment, which showed that particles can produce interference patterns characteristic of waves. The wave-particle duality is a fundamental aspect of quantum mechanics and is described by the de Broglie hypothesis, which states that any particle with momentum \( p \) has an associated wavelength \( \lambda = \frac{h}{p} \).
Uncertainty Principle
The uncertainty principle, formulated by Werner Heisenberg in 1927, states that certain pairs of physical properties, such as position and momentum, cannot be simultaneously measured with arbitrary precision. The principle is mathematically expressed as \( \Delta x \Delta p \geq \frac{h}{4\pi} \), where \( \Delta x \) and \( \Delta p \) are the uncertainties in position and momentum, respectively. The uncertainty principle highlights the intrinsic limitations of measurements in the quantum realm and challenges the deterministic nature of classical physics.
Quantum Superposition
Quantum superposition is the principle that a quantum system can exist in multiple states simultaneously until it is measured. This concept is famously illustrated by Schrödinger's cat thought experiment, in which a cat in a sealed box is simultaneously alive and dead until observed. Quantum superposition is a key feature of quantum mechanics and underlies phenomena such as quantum entanglement and quantum computing.
Applications of the Quantum Hypothesis
The quantum hypothesis has led to numerous technological advancements and applications in various fields, including electronics, computing, and medicine.
Quantum Electronics
Quantum electronics is the study and application of electronic devices that operate based on quantum mechanical principles. One of the most significant applications is the transistor, which is the fundamental building block of modern electronic devices. Transistors rely on the quantum behavior of semiconductors to amplify and switch electronic signals, enabling the development of computers, smartphones, and other digital technologies.
Quantum Computing
Quantum computing leverages the principles of quantum superposition and entanglement to perform computations that are infeasible for classical computers. Quantum computers use qubits instead of classical bits, allowing them to represent and process multiple states simultaneously. This parallelism enables quantum computers to solve complex problems, such as factoring large numbers and simulating molecular interactions, much more efficiently than classical computers.
Quantum Cryptography
Quantum cryptography exploits the principles of quantum mechanics to secure communication channels. One of the most well-known applications is quantum key distribution (QKD), which allows two parties to share a secret key with guaranteed security. QKD relies on the fact that any attempt to eavesdrop on the quantum communication channel will disturb the quantum states, revealing the presence of an intruder. This technology has the potential to revolutionize secure communication and data protection.
Challenges and Controversies
Despite its success, the quantum hypothesis and quantum theory have faced several challenges and controversies over the years.
Interpretations of Quantum Mechanics
One of the most debated aspects of quantum mechanics is its interpretation. The Copenhagen interpretation, formulated by Niels Bohr and Werner Heisenberg, is the most widely accepted interpretation, positing that quantum systems do not have definite properties until measured. However, alternative interpretations, such as the many-worlds interpretation and Bohmian mechanics, offer different perspectives on the nature of reality and the role of the observer in quantum measurements.
Quantum Measurement Problem
The quantum measurement problem concerns the question of how and why the act of measurement causes a quantum system to transition from a superposition of states to a single, definite state. This problem is closely related to the concept of wavefunction collapse and remains an open question in the foundations of quantum mechanics. Various interpretations and theories, such as decoherence and objective collapse models, have been proposed to address this issue, but no consensus has been reached.
Future Directions
The quantum hypothesis continues to inspire new research and developments in physics and technology. Emerging fields, such as quantum information science and quantum materials, hold promise for further advancements and applications.
Quantum Information Science
Quantum information science explores the processing, transmission, and storage of information using quantum systems. This interdisciplinary field encompasses quantum computing, quantum communication, and quantum sensing. Researchers are investigating new algorithms, protocols, and technologies to harness the power of quantum mechanics for information processing and secure communication.
Quantum Materials
Quantum materials are materials whose properties are governed by quantum mechanical effects. These materials exhibit unique behaviors, such as high-temperature superconductivity, topological insulators, and quantum spin liquids. Understanding and manipulating quantum materials could lead to breakthroughs in energy storage, electronic devices, and other technologies.
See Also
- Quantum Mechanics
- Photoelectric Effect
- Wave-Particle Duality
- Uncertainty Principle
- Quantum Superposition
- Quantum Computing
- Quantum Cryptography
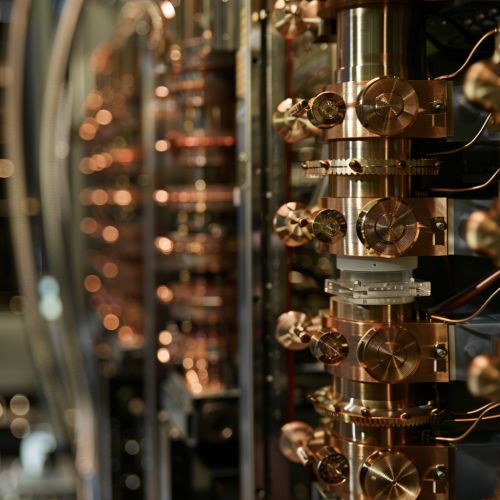
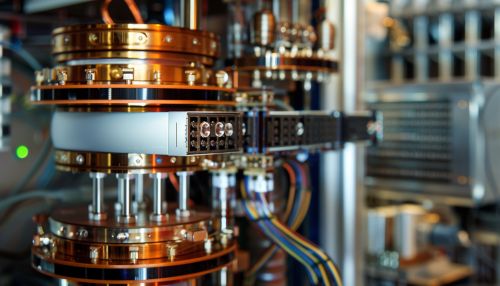