Quantum Dots Synthesis: Difference between revisions
(Created page with "== Introduction == Quantum dots (QDs) are semiconductor nanocrystals that exhibit unique optical and electronic properties due to quantum confinement effects. These properties make them highly valuable in various applications, including quantum computing, medical imaging, solar cells, and light-emitting diodes (LEDs). The synthesis of quantum dots is a complex process that requires precis...") |
No edit summary |
||
Line 17: | Line 17: | ||
The size and shape of the quantum dots can be controlled by adjusting the reaction temperature, time, and the concentration of precursors and ligands. | The size and shape of the quantum dots can be controlled by adjusting the reaction temperature, time, and the concentration of precursors and ligands. | ||
[[Image:Detail-97545.jpg|thumb|center|Laboratory setup for quantum dots synthesis, showing glassware, heating mantle, and chemical reagents.|class=only_on_mobile]] | |||
[[Image:Detail-97546.jpg|thumb|center|Laboratory setup for quantum dots synthesis, showing glassware, heating mantle, and chemical reagents.|class=only_on_desktop]] | |||
==== Hot-Injection Method ==== | ==== Hot-Injection Method ==== |
Latest revision as of 10:51, 8 August 2024
Introduction
Quantum dots (QDs) are semiconductor nanocrystals that exhibit unique optical and electronic properties due to quantum confinement effects. These properties make them highly valuable in various applications, including quantum computing, medical imaging, solar cells, and light-emitting diodes (LEDs). The synthesis of quantum dots is a complex process that requires precise control over size, shape, and composition to achieve the desired properties.
Synthesis Methods
The synthesis of quantum dots can be broadly categorized into two main approaches: chemical synthesis and physical synthesis. Each method has its own advantages and limitations, and the choice of method depends on the specific requirements of the application.
Chemical Synthesis
Chemical synthesis methods are widely used for producing high-quality quantum dots with controlled size and shape. The most common chemical synthesis methods include:
Colloidal Synthesis
Colloidal synthesis is the most popular method for producing quantum dots. It involves the reaction of precursors in a solvent at high temperatures to form nanocrystals. The key steps in colloidal synthesis are:
1. **Nucleation:** The initial formation of small clusters of atoms or molecules. 2. **Growth:** The enlargement of these clusters into nanocrystals. 3. **Capping:** The stabilization of nanocrystals with organic ligands to prevent aggregation.
The size and shape of the quantum dots can be controlled by adjusting the reaction temperature, time, and the concentration of precursors and ligands.
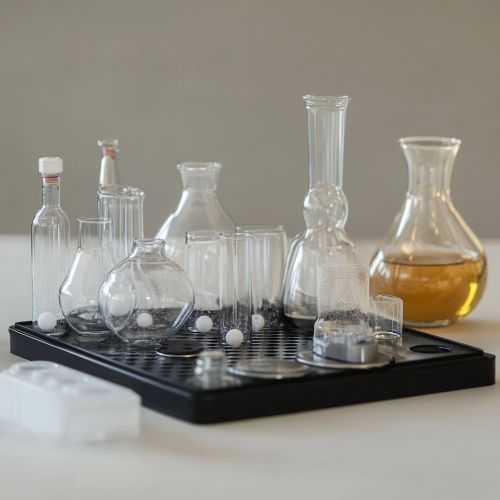
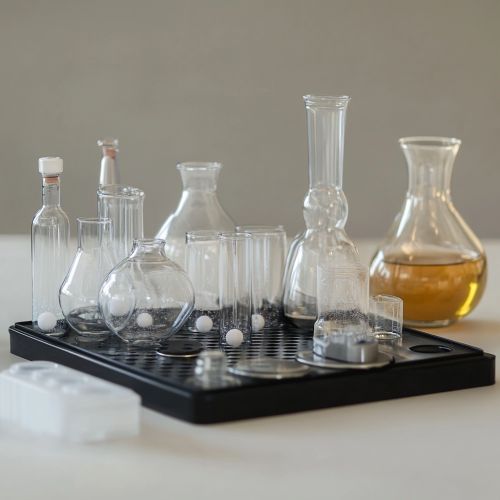
Hot-Injection Method
The hot-injection method is a variation of colloidal synthesis where precursors are rapidly injected into a hot solvent. This method allows for better control over the nucleation and growth processes, resulting in more uniform quantum dots. The key parameters in the hot-injection method are the injection temperature, the rate of injection, and the concentration of precursors.
Solvothermal and Hydrothermal Synthesis
Solvothermal and hydrothermal synthesis involve the use of high-pressure and high-temperature conditions to produce quantum dots. In solvothermal synthesis, an organic solvent is used, while in hydrothermal synthesis, water is the solvent. These methods are particularly useful for synthesizing quantum dots of materials that are difficult to produce using other methods.
Physical Synthesis
Physical synthesis methods involve the physical manipulation of materials to produce quantum dots. These methods include:
Molecular Beam Epitaxy (MBE)
Molecular beam epitaxy is a highly controlled method for producing quantum dots by depositing atoms or molecules onto a substrate in a vacuum environment. This method allows for precise control over the composition and thickness of the quantum dots, making it ideal for applications in quantum dot lasers and quantum dot solar cells.
Electron Beam Lithography (EBL)
Electron beam lithography is a technique used to create nanoscale patterns on a substrate by exposing it to a focused beam of electrons. This method can be used to produce quantum dots with precise shapes and sizes, making it suitable for applications in quantum dot transistors and quantum dot memory devices.
Ion Implantation
Ion implantation involves the introduction of ions into a substrate to create quantum dots. This method allows for precise control over the location and concentration of quantum dots, making it useful for applications in quantum dot sensors and quantum dot photodetectors.
Characterization of Quantum Dots
The characterization of quantum dots is essential to ensure that they possess the desired properties for specific applications. Common characterization techniques include:
Transmission Electron Microscopy (TEM)
Transmission electron microscopy is used to visualize the size, shape, and structure of quantum dots at the atomic level. TEM provides high-resolution images that can reveal defects and other structural features.
X-ray Diffraction (XRD)
X-ray diffraction is used to determine the crystal structure and phase composition of quantum dots. XRD patterns provide information about the lattice parameters and crystallinity of the quantum dots.
Photoluminescence (PL) Spectroscopy
Photoluminescence spectroscopy is used to study the optical properties of quantum dots. PL spectra provide information about the emission wavelength, quantum yield, and the presence of defects or impurities.
Absorption Spectroscopy
Absorption spectroscopy is used to measure the absorption properties of quantum dots. The absorption spectrum provides information about the bandgap energy and the size distribution of the quantum dots.
Applications of Quantum Dots
Quantum dots have a wide range of applications due to their unique optical and electronic properties. Some of the key applications include:
Medical Imaging
Quantum dots are used as fluorescent probes in medical imaging due to their bright and stable emission. They can be conjugated with biomolecules to target specific cells or tissues, enabling high-resolution imaging of biological processes.
Solar Cells
Quantum dots are used in solar cells to improve their efficiency by extending the absorption range of the solar spectrum. Quantum dot solar cells have the potential to achieve higher power conversion efficiencies compared to traditional solar cells.
Light-Emitting Diodes (LEDs)
Quantum dots are used in LEDs to produce high-quality light with tunable colors. Quantum dot LEDs offer advantages such as high brightness, low power consumption, and long lifetimes.
Quantum Computing
Quantum dots are used as qubits in quantum computing due to their ability to confine and manipulate individual electrons. Quantum dot qubits offer advantages such as long coherence times and scalability.
Challenges and Future Directions
Despite the significant progress in the synthesis and application of quantum dots, several challenges remain. These include:
- **Scalability:** Developing scalable synthesis methods to produce large quantities of high-quality quantum dots.
- **Stability:** Improving the stability of quantum dots to prevent degradation over time.
- **Toxicity:** Addressing the potential toxicity of quantum dots, particularly those containing heavy metals such as cadmium.
Future research is focused on developing new synthesis methods, exploring novel materials, and improving the performance and safety of quantum dots for various applications.
See Also
References
- Alivisatos, A. P. (1996). "Semiconductor Clusters, Nanocrystals, and Quantum Dots". Science. 271 (5251): 933–937.
- Murray, C. B., Norris, D. J., & Bawendi, M. G. (1993). "Synthesis and Characterization of Nearly Monodisperse CdE (E = S, Se, Te) Semiconductor Nanocrystallites". Journal of the American Chemical Society. 115 (19): 8706–8715.