Quantum dot solar cell: Difference between revisions
(Created page with "== Introduction == A '''quantum dot solar cell''' (QDSC) is a type of solar cell that employs quantum dots as the photovoltaic material. Quantum dots are semiconductor nanocrystals that exhibit quantum mechanical properties. These properties enable quantum dots to have tunable bandgaps, which can be adjusted by changing their size. This tunability offers significant potential for enhancing the efficiency of solar cells by optimizing the absorption of the...") |
No edit summary |
||
Line 74: | Line 74: | ||
Quantum dot solar cells represent a promising technology for the future of photovoltaics, offering the potential for high efficiency and versatile applications. Ongoing research and development efforts are focused on addressing the challenges and unlocking the full potential of this innovative technology. | Quantum dot solar cells represent a promising technology for the future of photovoltaics, offering the potential for high efficiency and versatile applications. Ongoing research and development efforts are focused on addressing the challenges and unlocking the full potential of this innovative technology. | ||
[[Image:Detail-92745.jpg|thumb|center|Close-up image of a quantum dot solar cell under sunlight.|class=only_on_mobile]] | |||
[[Image:Detail-92746.jpg|thumb|center|Close-up image of a quantum dot solar cell under sunlight.|class=only_on_desktop]] | |||
== See Also == | == See Also == |
Latest revision as of 21:49, 20 June 2024
Introduction
A quantum dot solar cell (QDSC) is a type of solar cell that employs quantum dots as the photovoltaic material. Quantum dots are semiconductor nanocrystals that exhibit quantum mechanical properties. These properties enable quantum dots to have tunable bandgaps, which can be adjusted by changing their size. This tunability offers significant potential for enhancing the efficiency of solar cells by optimizing the absorption of the solar spectrum.
Background
Quantum dots are nanometer-sized semiconductor particles that have unique optical and electronic properties due to quantum confinement effects. These effects arise when the size of the quantum dot is smaller than the exciton Bohr radius, leading to discrete energy levels. The ability to tune the bandgap of quantum dots by altering their size makes them highly attractive for various applications, including solar cells.
Working Principle
The working principle of quantum dot solar cells is similar to that of traditional photovoltaic cells, but with some key differences due to the unique properties of quantum dots. When light is absorbed by a quantum dot, an electron-hole pair (exciton) is created. The exciton can then be separated into free charge carriers (electrons and holes) that can be collected to generate electric current.
Absorption and Charge Separation
Quantum dots can absorb photons with energies greater than their bandgap. The absorbed energy excites an electron from the valence band to the conduction band, leaving behind a hole in the valence band. The electron and hole can be separated by an electric field or by the energy gradient at the interface of different materials within the solar cell.
Multiple Exciton Generation
One of the most promising features of quantum dots is their ability to undergo multiple exciton generation (MEG). MEG is a process where a single high-energy photon generates multiple electron-hole pairs, potentially increasing the efficiency of the solar cell beyond the Shockley-Queisser limit, which is the theoretical maximum efficiency of a single-junction solar cell.
Materials and Fabrication
Quantum dot solar cells can be fabricated using various materials and techniques. The choice of materials and fabrication methods can significantly impact the performance and efficiency of the solar cells.
Quantum Dot Materials
Common materials used for quantum dots in solar cells include cadmium selenide (CdSe), lead sulfide (PbS), and indium phosphide (InP). Each material has its own advantages and challenges, such as toxicity, stability, and bandgap tunability.
Fabrication Techniques
Several techniques are used to fabricate quantum dot solar cells, including colloidal synthesis, chemical vapor deposition (CVD), and molecular beam epitaxy (MBE). Colloidal synthesis is a popular method due to its simplicity and scalability. It involves the chemical synthesis of quantum dots in a solution, which can then be deposited onto a substrate to form a thin film.
Device Architectures
Quantum dot solar cells can be designed in various architectures, each with its own advantages and challenges.
Schottky Junction Solar Cells
In Schottky junction solar cells, a layer of quantum dots is sandwiched between a metal and a semiconductor. The metal forms a Schottky barrier with the quantum dots, facilitating charge separation and collection. This architecture is relatively simple but may suffer from lower efficiency due to recombination losses.
p-n Junction Solar Cells
In p-n junction solar cells, quantum dots are used to form either the p-type or n-type layer, or both. The p-n junction creates an electric field that helps separate and collect charge carriers. This architecture can achieve higher efficiencies but requires precise control over the doping and placement of quantum dots.
Bulk Heterojunction Solar Cells
Bulk heterojunction solar cells use a blend of quantum dots and a conducting polymer to form a composite layer. This architecture provides a large interface area for charge separation and can be fabricated using solution-based techniques. However, achieving optimal morphology and charge transport remains a challenge.
Performance and Efficiency
The performance of quantum dot solar cells is evaluated based on several key parameters, including power conversion efficiency (PCE), open-circuit voltage (Voc), short-circuit current density (Jsc), and fill factor (FF).
Power Conversion Efficiency
The power conversion efficiency is the ratio of the electrical power output to the incident solar power input. Quantum dot solar cells have demonstrated efficiencies exceeding 10%, with ongoing research aiming to further improve this metric.
Open-Circuit Voltage and Short-Circuit Current Density
The open-circuit voltage is the maximum voltage the solar cell can produce when not connected to an external circuit, while the short-circuit current density is the current produced when the cell is short-circuited. Both parameters are influenced by the material properties and device architecture.
Fill Factor
The fill factor is a measure of the quality of the solar cell's output characteristics. It is the ratio of the maximum power output to the product of Voc and Jsc. A higher fill factor indicates better performance.
Challenges and Future Directions
Despite their potential, quantum dot solar cells face several challenges that need to be addressed to achieve commercial viability.
Stability and Toxicity
The stability of quantum dot solar cells is a major concern, as quantum dots can degrade over time due to environmental factors such as oxygen and moisture. Additionally, some quantum dot materials, such as cadmium-based compounds, are toxic and pose environmental and health risks.
Charge Transport and Recombination
Efficient charge transport and minimizing recombination losses are critical for achieving high performance. Researchers are exploring various strategies, such as surface passivation and the use of core-shell quantum dots, to improve charge transport and reduce recombination.
Scalability and Manufacturing
Scaling up the production of quantum dot solar cells while maintaining high quality and low cost is a significant challenge. Advances in fabrication techniques and materials are needed to enable large-scale manufacturing.
Applications
Quantum dot solar cells have potential applications in various fields due to their unique properties.
Building-Integrated Photovoltaics
Quantum dot solar cells can be integrated into building materials, such as windows and facades, to generate electricity while maintaining transparency and aesthetic appeal.
Portable and Wearable Electronics
The flexibility and lightweight nature of quantum dot solar cells make them suitable for use in portable and wearable electronic devices, providing a sustainable power source.
Space Applications
The tunable bandgap and high efficiency of quantum dot solar cells make them attractive for space applications, where maximizing power output and minimizing weight are critical.
Conclusion
Quantum dot solar cells represent a promising technology for the future of photovoltaics, offering the potential for high efficiency and versatile applications. Ongoing research and development efforts are focused on addressing the challenges and unlocking the full potential of this innovative technology.
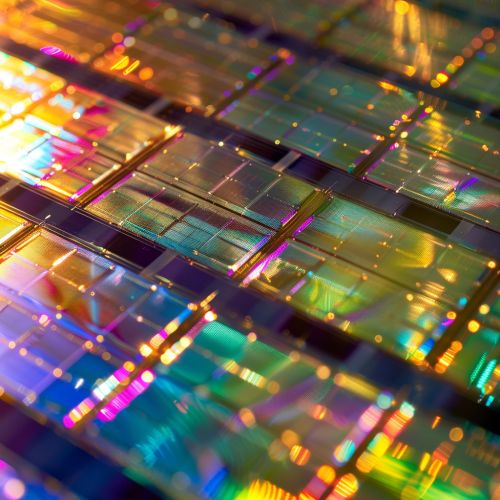
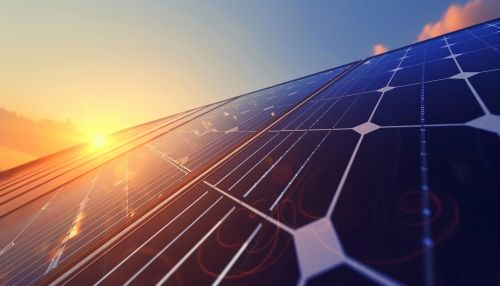